|
|
|
ABSTRACT |
This study examined the effects of poles when walking on the rate of perceived exertion (RPE), physiological and kinematics parameters, and upon the mean ratio between locomotor and respiratory rhythms. Twelve healthy male and female volunteers, aged 22 to 49 years old, completed on a motorized treadmill in a counterbalanced randomized order 12 walking trials for 10 min at an individually preferred walking speed, with three grades (horizontal level, uphill or downhill with a slope of 15%), with and without hiking poles and a load carriage of 15% of body mass. During all testing sessions, heart rate (HR), oxygen consumption (VO2), ventilation (VE), tidal volume (VT), breathing frequency (Bf), and stride frequency were recorded continuously during the last 5-min of each trial. At the end of each trial, subjects were asked to give RPE. Energy cost (EC) and VE increased significantly with the grade (-15% < 0% < +15%) and with the carrying load. VT was significantly less important with hiking poles, while Bf was significantly more elevated. VO2 and EC increased (p < 0.05) with the use of the hiking poles only during the downhill trials. No significant effect of poles was observed on HR, RPE, and preferred walking speed. The average ratio between the locomotor and respiratory frequencies was significantly influenced by the three experimental factors tested. There was a significant relationship between average ratio of leg movement per breath and EC of walking among all conditions (r = 0.83, n = 12). These results suggest that the use of the hiking poles had a significant influence on the respiratory and energetic responses only during downhill walking. |
Key words:
Energy cost, grades, hiking poles, respiration, nordic-walking
|
Key
Points
- Energetic cost, respiratory responses, stride rate, respiratory to cycle rate ratio were significantly influenced by the use of hiking poles according to the grade at self-selected walking speed.
- Hiking poles induced an increase in respiratory frequency, VE and energetic cost during downhill, while little changes were observed during level and uphill terrain.
- The original results obtained in downhill necessitate supplementary studies in the field in order to confirm these first tendencies on treadmill.
|
Since ten years, the use of hiking poles in recreational walking is becoming increasingly popular, especially among elderly. Their use is being justified by reduced stress on lower limbs and spine, as well as by increased balance, ease of walking, reduced fatigue and additional exercising of the shoulders and arms musculature (Blake and Fergusson, 1993; Bohne and Abendroth-Smith, 2007; Schwameder et al., 1999). In spite of the importance of these factors, some questions regarding the use and effect of hiking poles remain. The questions like, when to use hiking poles, do they affect the energy expenditure of walking, are still being unknown. Significant higher values for oxygen uptake (VO2) were measured at 6.5 and 7.2 km·h-1 with 8 and 7% incline, respectively for Nordic walking compared to walking without poles (Schiffer et al., 2006). In the same way, Rodgers et al., 1995 reported that hiking poles significantly increased VO2 by 3 ml·min-1·kg-1 as well as heart rate (HR) by 11 beats.min-1 and overall energy consumption (in kcals) by some 20 % in comparison with walking at the same speed (6-7.5 km·h-1) without poles on a treadmill at 0% grade. Surprisingly, the effort required for the two forms of walking was perceived to be about the same (Rodgers et al., 1995; Jacobson et al., 2000). In contrast, Jacobson et al., 2000 reported that hiking poles during inclined walking with a 15 kg load carriage does not alter the associated energy cost (EC) despite the added weight. Differences between the three aforementioned studies may be attributed to the contrast in arm swing (vigorous vs. smooth), load carriage (without vs. with a load of 15 kg), and treadmill incline (0% vs. 10-25%). As suggested by Jacobson et al., 2000, it is likely that amplified arm swing while using hiking poles increases energy expenditure when contrasts with a natural and smooth arm action. To date, we do not know whether the degree of exertion while walking with hiking poles change for different grades (uphill, downhill, and level terrain) at preferential walking speed with a load carriage (i.e., typical conditions that we can observe during outdoor recreation). In the study of Jacobson et al., 2000, testing sessions were performed during a progressive and continuous treadmill protocol with short periods (1 or 2 min) for each grade condition (from +10 to 25% grades). Moreover, all subjects completed trials of treadmill walking at the same speed (Jacobson et al., 2000; Rodgers et al., 1995) and with the same load (15 kg in Jacobson et al., 2000). Under field conditions, individuals walk usually at their preferred walking speed, also called comfortable gait speed. There is evidence to suggest that an individual’s preferred walking speed is at or near his or her most economical speed (Pearce et al., 1983). Thus, the possibility exists that the reason for concluding that the energy expenditure is affected by hiking poles, may be caused by the imposed walking speed. At faster walking speeds, the increased pumping action of the arms, which is necessary to achieve and maintain some unaccustomed speeds, affects EC. Given the range of speeds, grades, and protocols used by previous investigators, the objective of this research was to obtain comparative data for metabolic, cardiac and ventilatory variables, stride rate, and rating of perceived exertion (RPE) for people walking at a constant self-selected speed for different grades. It was hypothesized that the exertion of walking with hiking poles would not differ from that of walking without poles at preferred walking speed for different grades. Transition from rest to dynamic movement is characterized by a breathing pattern which is energetically optimum for the given ventilatory demand. It has been suggested that respiratory frequency in humans is dependant on limb movement frequency (Bechbache and Duffin, 1977; Rassler and Kohl, 1996; Siegmund et al., 1999). During walking, individuals move their arms contralaterally with the legs, and the breathing pattern accompanying such dynamic arm exercises may be complicated by phasic impulses from the working limbs. The arm action plus upper body motion with hiking poles according to the grade may influence the breathing pattern of walkers and the subsequent effect on EC. The second purpose of this study was to examine the influence of average ratio of leg movement per breath on EC of walking. It was hypothesized that using the poles would result in a shift in the relationship between EC and the average ratio of leg movement per breath.
SubjectsFive male and seven female subjects between the ages of 22 and 49 years (Table 1) volunteered for this study. Subjects filled out a health history questionnaire and signed a consent form. Subjects were verbally informed about the experimental protocol and applied methods, but not on the purpose of the study. Subjects were instructed on the use of hiking poles on a treadmill with different walking speeds and grades before participating in any testing. Practice sessions were held two times per week, totaling at least 30-min trials. The subjects were all healthy and practiced regularly Nordic walking for leisure. The study procedures complied with the Declaration of Helsinki for human experimentation and were approved by the local ethics committee.
Experimental protocolEach of the 12 subjects performed the 3 x 2 x 2 (grades x poles x load carriage) different exercise trials (on two different days separated by at least 48 h) in a counterbalanced randomized order at an individually preferred walking speed (PS). Subjects walked on a motor driven treadmill (S2500, HEF Techmachine, Andrézieux Bouthéon, France) at grades of 0, ± 15%, carrying or not a backpack loaded to 15% body mass, and with and without hiking poles. Each exercise trial was fixed to 10 min, where the first 5-min was devoted to warm-up, accommodation, and selection of PS. The last 5-min was dedicated to data collection period when the subject was at the self-selected PS for a given condition. On completing each 10-min trial (6 repetitions in one day), subjects were disconnected from the metabolic system, after which they rested to allow their HR to come within 5 beats·min-1 of resting values. At the end of each trial, subjects were asked to perceive their rate of exertion (RPE) on the 6-20 graded scale proposed by Borg, 1982. The PS (in km·h-1) was determined for each condition in all subjects according to the method proposed by Martin et al., 1992. First, starting with the treadmill speed at 1.5 km·h-1, walking speed was slowly increased until the individual subjectively identified his or her preferred walking speed. This speed was then maintained for 1 min, after which the subject was again asked to evaluate the speed. Speed adjustments were subsequently made according to subject directive. Then, this procedure was repeated except that the treadmill speed was started from 8.0 km·h-1 and gradually reduced. The preferred walking speed was finally determined as the average of the two subjective estimates of speed (within 0.4 km·h-1) with the instructions to find a comfortable walking pace. The load of 15% of body mass was placed in a commercially made backpack (Salomon, Inc.) equipped with adjustable sternum strap, hip belt, and load lifters. Traditional hiking poles (model Tibet antishock, McKinley, Italy, mass of 325 g each pole) were individually fit for each subject with adjustable, telescopic sections and wrist straps by taking into account the elbow joint angle at plant (i.e., 90° while the pole was held in a vertical position and in contact with the ground).
Materials Gas exchange and ventilatory parameters were collected breath-by-breath during all trials by means of a portable (mass of 450 g) metabolic system (Cosmed K4b2, Rome, Italy). HR was monitored continuously using a wireless HR monitor (Polar, Kempele, Finland), and was synchronized to ventilatory and gas signals. Gas analyzers were calibrated before each test with ambient air (O2: 20.93 % and CO2: 0.03 %) and a gas mixture of known composition (O2: 16.00 % and CO2: 5.00 %). The facemask was equipped with a low-resistance, bidirectional digital turbine (28-mm diameter) that was calibrated before each test with a 3-L syringe (Hans Rudolph Inc, Dallas, USA). Inspiratory and expiratory flow was measured continuously by a nasal thermistance (SS6L temperature transducer BSL, Biopac Systems, Inc., Santa Barbara, USA) attached just under the nostril of the subject. Finally, a mechano-electrical goniometer was fixed on the right knee of all subjects in order to record the stride rate during walking trials. These two analogical sensors were continuously recorded and synchronized at 1000 Hz during the last 5 min of each trial by means of the Biopac MP30 unit (Biopac Systems, Inc., Santa Barbara, USA).
AnalysisVentilatory and gas exchange variables were averaged during the last 2-min of each trial after metabolic steady state achievement and constant walking pace. Minute ventilation (VE, l·min-1), breathing frequency (Bf, cycles·min-1), tidal volume (VT, l), HR (beats·min-1), VO2 (ml·min-1·kg-1) and respiratory exchange ratio (RER) were then determined. In our design, VO2 was representative of the total EC. As a result, the aerobic EC (mlO2·kg-1·m-1) of walking was calculated as the quotient of steady-state VO2 divided by the walking speed. Finally, to evaluate the relationship between stride rate and breathing frequency, we estimated the average ratio of locomotor movement (stride rate) to breathing frequency (inspiratory and expiratory flow) during the last 2 min of each 10-min trial.
Statistical analysisAll data are presented as means ± SD. Significant differences for grade, load carriage, and hiking poles were calculated by analysis of variance with repeated measurements [3 (grade) x 2 (hiking poles) x 2 (load carriage)], and were completed with the post-hoc Scheffé test. Comparisons were made on the following dependent variables: respiratory parameters (VE, VT, Bf, VO2), stride frequency, HR, EC, RPE and the mean ratio of stride rate per breath. A linear regression was performed to show relationship between EC and average ratio of locomotor movement to breathing frequency. Significativity level was fixed to p < 0.05. The software used was Statistica 7.1 (Statsoft Inc., Maisons-Alfort, France).
All subjects carried out all experimental conditions. Only one recording was not performed with the portable metabolic system due to technical problem. Results are therefore presented either for 11 or 12 subjects accordingly. There was a significant effect of the grade on PS with a rank order as level (4.7 ± 0.6 km·h-1) > downhill (4.5 ± 0.7 km·h-1) > uphill (4.1 ± 0.6 km·h-1). Post-hoc tests indicated that PS was significantly lower during uphill compared to downhill and level (p < 0.05). There were neither hiking poles nor load carriage effects on PS.
Energy expenditureRER values were only influenced significantly by grade. Post-hoc tests showed that RER was significantly lower (p < 0.05) during level (0.88 ± 0.06) compared with downhill (0.92 ± 0.10) and uphill (0.96 ± 0.04) trials. VO2 values were significantly higher with carrying load [F(1, 10) = 23.5, p < 0.001], with poles [F(1, 10) = 6.1, p < 0.05] and during uphill [F(2, 20) = 217.1, p < 0.001]. An interaction effect (poles x grade) was observed [F(2, 20) = 16.6, p < 0.001]. Post hoc tests revealed that there were no significant differences between trials with and without poles during either level or uphill terrain. However, VO2 values were significantly higher with the use of hiking poles during downhill walking (+ 19%, p < 0.05). Results for EC displayed in Figure 1 were the same than those for VO2 due to the small differences in PS. Values of EC were significantly higher with carrying external load [F(1, 10) = 17.1, p < 0.001], with poles [F(1, 10) = 5.62, p < 0.05] and during uphill [F(2, 20) = 296.5, p < 0.001]. As indicated in Figure 1, poles x grade interaction showed significantly higher EC values with poles during downhill walking (+ 23%, p < 0.05). As expected, interaction effect of load x grade showed a significantly higher EC with load during uphill walking (+ 13%, p < 0.05). HR was significantly higher during uphill compared to level (+ 36%, p < 0.05) and downhill terrain (+ 52%, p < 0.05), and with carrying external load (+ 8%, p < 0.05) than without. HR was not influenced by the use of poles.
Ventilatory variablesValues of VE were significantly higher with carrying load [F(1, 10) = 24.5, p < 0.001] and during uphill [F(2, 20) = 113.9, p < 0.001]. As displayed in Figure 2, an interaction effect between poles x grade was observed [F(2, 20) = 9.95, p < 0.001]. Post-hoc tests showed that with poles, VE was significantly higher (p < 0.05) during downhill and level trials. Carrying load of 15 % body mass [F(1, 10 = 14.7, p < 0.01], the no use of hiking poles [F(1, 10) = 6.3, p < 0.05], and downhill terrain [F(2, 20) = 264.1, p < 0.001] increased VT values. Moreover, VT with carrying load was significantly higher with poles by 7% than without; this difference disappeared without load carriage. Importantly, VT was significantly lower by 9 % with poles than without during uphill (Figure 2B). Values of Bf were significantly higher during trials with poles [F(1, 10) = 22.8, p < 0.001] and with carrying load [F(1, 10) = 23.5, p < 0.001]. Using poles induced significantly higher Bf values regardless the grade, but this difference was only significant during downhill terrain (p < 0.05, Figure 2C).
Stride rate and mean ratio of frequency of stride per breathStride rate was significantly influenced by grade, poles and by the interaction effect between grade x poles (p < 0.001). Stride rate values were lower with hiking poles than without (mean values of 0.88 vs. 0.92 Hz, respectively) and increased according to the following rank order: uphill < level < downhill. The lowest stride rate values occurred with hiking poles during uphill terrain (0.80 Hz, p < 0.05). The averaged ratio between rhythms of locomotion and breathing was increased with compared to without carrying load [F(1, 11) = 23.1, p < 0.01)], poles [F(1, 11) = 53.1, p < 0.001], and according to the increasing grade [F(2, 22) = 26.1, p < 0.001]. An interaction effect between experimental conditions load x hiking poles x grade was observed [F(2, 22) = 3.7, p < 0.05]. There was a significant relationship between the average ratio of locomotor movement to breathing frequency and EC of walking among all experimental conditions (r = 0.83, n = 12, SEE = 0.08, p < 0.001, Power of 0.94). Figure 3 was generated from the data set to separate walking conditions with and without poles.
Perceived exertionThe RPE values were significantly higher with the grade [F(1, 11) = 68.9, p < 0.001] and the load carriage [F(2, 22) = 64.8, p < 0.001]. An interaction effect load x grade was observed [F(2, 22) = 9.1, p < 0.001] where the influence of the load was more pronounced during uphill compared with downhill and level terrain. There was no significant difference in RPE with (10.2 ± 2.3) compared to without (9.9 ± 2.6) poles.
This study aimed to compare physiological responses (VO2, HR, VE, VT and Bf) and RPE during walking exercise trials on different grades (0% and ± 15%), carrying or not a backpack loaded to 15% body mass, and with and without hiking poles. The main results showed that the poles influenced significantly the respiratory responses (VE and its determinants, VT and Bf) and aerobic EC according to the grade. As discussed below, the results of the present study are more or less in agreement on many points with the few studies dealing about influence of hiking poles on energy expenditure (Jacobson et al., 2000; Knight et al., 2000; Porcari et al., 1997; Rodgers et al., 1995; Schiffer et al., 2006). Experimental design in these previous studies induced a fatigue effect over the tests and imposed a same walking speed for all subjects. More important, only the rising profile was experimented in these studies, underlining some original results of the present study on level and downhill terrain. In addition, we chose to use a preferential speed so that the subjects walked at their comfort speed for each condition as on field conditions; then EC of walking was determined. However, one limitation in the present study may come from Nordic walking on a treadmill probably not so representative that free walking with hiking poles on the field as optimal pole plant with a fixed ground contact is impaired by the moving walking belt. In the present study, familiarization sessions were realized. All the subjects showed a temperate and fluid swaying of the upper body, without excessive movements.
Energy expenditureIn contrast to the results of Knight and Caldwell, 2000 that noted an 5.6% increase in HR, our study did not reveal some influence of using walking poles on HR corroborating nevertheless the results of Jacobson et al., 2000. Further, poles had no effect on HR in the present study regardless the grade. This is in disagreement with Rodgers et al. (1995) but in line with the recent studies of Jacobson et al., 2000 and Schiffer et al., 2006. In this study, HR significantly increased only during the most constraining experimental conditions , i.e. with carrying load during uphill. The pattern of response of HR, considered as an indirect index of energy expenditure more easily quantifiable on the field, is however well different of VO2. Energy expenditure as estimated by VO2 and EC for similar PS was influenced significantly by using poles only in downhill trials (Figure 1.). The current data suggest that when the hiking poles are used without excessive movements, no additional energy expenditure occurs during uphill (Jacobson et al., 2000; Knight and Caldwell, 2000; present study) and level ground (present study) despite the added weight of the hiking poles (mass of 325 grams x 2). On motorized treadmill, as noticed by Jacobson et al., 2000, the ground-pole contacts are likely less important that on trails, suggesting a less use of the poles at the time of the propulsion. Another factor may be related to subjects’ walking speed in each study. The self-selected speeds by our subjects for all grades regardless the use of poles were less (~1 to 2 km.h-1) than those in the aforementioned studies. However the greatest difference in EC between the pole and no-pole conditions occurs at the slowest walking speeds (Porcari et al., 1997). Finally, the unexpected result in downhill (higher EC with poles) deserves further studies but may be due to some ventilatory responses related to mechanics of downhill walking.
Ventilatory response patternsConcerning the respiratory variables, we observed a crossed effect of the hiking poles x grade on VE. During level and uphill, there were no significant differences between the conditions with and without hiking poles (Figure 2). However, VE was significantly higher with poles during downhill. This result should be compared narrowly with those of VO2 and EC. Among the determining parameters of VE, Bf and VT presented opposite responses. For all conditions, Bf was significantly higher with poles than without. More specifically, interaction analysis (poles x grade) showed that Bf was higher only during downhill, while VT was unchanged. We conclude therefore that the use of hiking poles during downhill walking induces an increased Bf resulting in an increased VE (unchanged VT), and subsequent increase in VO2 and EC. Rassler and Kohl, 1996 had already observed that the major changes in VE during several walking conditions were explained primarily by Bf and not by VT responses. The increase in Bf may suggest that the propulsive action of the upper body could interact with the control of the ventilatory rhythm due to the proximity of the respiratory and locomotor muscles (Amazeen et al., 2001; Fabre et al., 2007). According to different Bf responses observed with grade (Figure 2), mechanics of downhill walking may be different from the other two walking trials.
Stride rate and mean ratio of frequency of stride per breathOur results showed that the grade, the poles and interaction of both (p < 0.001) significantly influenced the stride rate. Stride rate was the lowest with the poles during uphill (0.80 Hz, p < 0.05), suggesting that the optimization of the energy expenditure (EC was not modified during trials with and without the poles) may be linked up to a modification of the locomotor rhythm. Stride rate seems therefore to determine an optimal energetic speed (Pearce et al., 1983), as well as Bf is the main regulating control factor for the ventilatory system. The influences of the rhythms on the locomotor and respiratory systems during human locomotion have been studied extensively (Banzett et al., 1992; Bramble and Carrier, 1983; Fabre et al., 2007; Rassler and Kohl, 1996; Siegmund et al., 1999), and may be in favor of a movement economy. The average ratio between the locomotor and respiratory frequencies estimated on the last 2 min was significantly influenced by the three experimental factors tested in the present study. Our results showed clearly the influences of the slope, load carriage and use of poles on this ratio. The significant differences between the average ratio as a function of the grade (in particular during uphill), can be interpreted by the opposite changes in stride rate and Bf. Concerning the influence of the hiking poles, the proximity of the respiratory and locomotor muscles during the walking exercise with poles can explain the significantly higher average ratio with poles than without (Figure 3). The propulsive forces or the simple grip of the poles by the upper body would allow increasing the mechanical constraints of the locomotor and respiratory muscles (intercostal, abdominal muscles and diaphragm, pectoral, etc.). In final, these results show that the adjustment of a frequency to another is changed when we constrained the locomotion (e.g. additional poles, grades) and we noted accordingly a significant influence of average ratio of leg movement per breath on EC of walking (Figure 3) with and without poles as a function of grade.
Rate of perceived exertionOur results did not show significant influence of the use of the hiking poles on RPE. As underlined above with HR, RPE was significantly increased during uphill and with the load carriage. These results are therefore in contradiction with those of Jacobson et al., 2000 and Knight and Caldwell, 2000 that showed in their respective studies a significant decrease in RPE with poles. In the study of Jacobson et al., 2000, the difference can be explain by the fact that the transitions of a slope to another (10, 15, 20 and 25%) lasted of 1 to 10 min according to the slope, and were linked progressively (total time exercise of 15 min with an absolute additional load of 15 kg). In the present study the exercise periods for a given condition were of 5 min (with the goal to achieve a steady state) and intermittent (minimization of accumulated fatigue). The study of Knight and Caldwell, 2000 had a similar exercising time period than in the present study (60 min) but in a continuous way, and with an additional load of 30% of the body mass on a slope of 5°. Fatigue effect was therefore totally different among studies. In addition, only the effect of poles was tested in Knight and Caldwell, 2000.
In conclusion, our results show that energy expenditure and perceived exertion during uphill and level does not change significantly with the use of hiking poles at self-selected walking speed. Optimize caloric expenditure with poles while walking at submaximal self-selected speeds was verified in the context of our study during uphill and level conditions. Interestingly, downhill walking induced higher energy expenditure and ventilatory responses with than without poles. We proposed that at self-selected walking speed, walkers who want to use poles during downhill should adapt differently breathing and mechanics of locomotion to lower energy expenditure.
ACKNOWLEDGEMENTS |
We wish to thank for their help in data collection and technical assistance Sebastien Villard and Laura Barony. |
|
AUTHOR BIOGRAPHY |
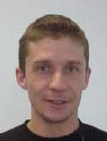 |
Stephane Perrey |
Employment: Prof. at the Department of Physical Training & Sports Engineering, Faculty of Sport Sciences, University of Montpellier I, France. |
Degree: PhD |
Research interests: Exercise physiology, acute and chronic cardiorespiratory and muscle responses of human to exercise, physiological responses of endurance and power athletes, and neuromuscular fatigue. |
E-mail: stephane.perrey@univ-montp1.fr |
|
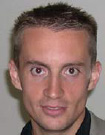 |
Nicolas Fabre |
Employment: Prof. at the Department of Sport Sciences, University of Pau, France. |
Degree: PhD |
Research interests: Exercise physiology, locomotor-respiratory coordination in human, physical training. |
E-mail: fabre.nicolas@tiscali.fr |
|
|
|
REFERENCES |
 Amazeen P.G., Amazeen E.L., Beek P.J. (2001) Coupling of breathing and movement during manual wheelchair propulsion. Journal of Experimental Psychology: Human Perception and Performance 27, 1243-1259.
|
 Banzett R.B., Mead J., Reid M.B., Topulos G.P. (1992) Locomotion in men has no appreciable mechanical effect on breathing. Journal of Applied Physiology 72, 1922-1926.
|
 Bechbache R.R., Duffin J. (1977) The entrainment of breathing frequency by exercise rhythm. Journal of Physiology 272, 553-561.
|
 Blake R.L., Ferguson H.J. (1993) Walking and hiking injuries. A one year follow-up study. Journal of the American Podiatric Medical Association 83, 499-503.
|
 Bohne M., Abendroth-Smith J. (2007) Effects of hiking downhill using trekking poles while carrying external loads. Medicine and Science in Sports and Exercise 39, 177-183.
|
 Borg G.AV. (1982) Psychological basis of perceived exertion. Medicine and Science in Sports and Exercise 14, 377-387.
|
 Bramble D.M., Carrier D.R. (1983) Running and breathing in mammals. Science 219, 251-256.
|
 Fabre N., Perrey S., Arbez L., Rouillon J.-D. (2007) Neuro-mechanical and chemical influences on locomotor respiratory coupling in humans. Respiratory Physiology & Neurobiology 155, 128-136.
|
 Jacobson B.H., Wright T., Dugan B. (2000) Load carriage energy expenditure with and without hiking poles during inclined walking. International Journal of Sports Medicine 21, 356-359.
|
 Knight C.A., Caldwell G.E. (2000) Muscular and metabolic costs of uphill backpacking: are hinking poles benefical ?. Medicine and Science in Sports and Exercise 32, 2093-2101.
|
 Martin P.E., Rothstein D.E., Larish D.D. (1992) Effects of age and physical activity status on the speed-aerobic demand relationship of walking. Journal of Applied Physiology 73, 200-206.
|
 Pearce M.E., Cnningham C.A., Dnner A.P., Rchnitzer P.A., Fllerton G.M., Hward J.H. (1983) Energy cost of treadmill and floor walking at self-selected paces. European Journal of Applied Physiology 52, 115-119.
|
 Porcari J.P., Hendrickson T.L., Walter P.R., Terrey L., Walsko G. (1997) The physiological responses to walking with and without Power Poles on treadmill exercise. Research Quality in Exercise and Sport 68, 161-166.
|
 Rassler B., Kohl J. (1996) Analysis of coordination between breathing and walking rhythms in humans. Respiration and Physiology 106, 317-327.
|
 Rodgers C.D., Vanheest J.L., Schachter C.L. (1995) Energy expenditure during submaximal walking with Exerstriders®. Medicine and Science iIn Sports and Exercise 27, 607-611.
|
 Schiffer T., Knicker A., Hoffman U., Harwig B., Hollmann W., Struder H.K. (2006) Physiological responses to nordic walking, walking and jogging. European Journal of Applied Physiology 98, 56-61.
|
 Schwameder H., Roithner R., Müller E., Niessen W., Raschner C. (1999) Knee joint forces during dowhnill walking with hiking poles. Journal of Sports Science 17, 969-978.
|
 Siegmund C.P., Edwards M.R., Moore K.S., Tiessen D.A., Sanderson D.J., McKenzie D.C. (1999) Ventilation and locomotion coupling in varsity male rowers. Journal of Applied Physiology 87, 233-242.
|
|
|
|
|
|
|