|
|
|
ABSTRACT |
The purpose of this study was to assess the effect of three different body positions on HRV measures following short-term submaximal exercise. Thirty young healthy males performed submaximal cycling for five minutes on three different occasions. Measures of HRV were obtained from 5-min R to R wave intervals before the exercise (baseline) and during the last five minutes of a 15 min recovery (post-exercise) in three different body positions (seated, supine, supine with elevated legs). Measures of the mean RR normal-to-normal intervals (RRNN), the standard deviation of normal-to-normal intervals (SDNN), the root mean square of successive differences (RMSSD) and the low-frequency (LF) and the high-frequency (HF) spectral power were analyzed. Post-exercise RRNN, RMSSD were significantly higher in the two supine positions (p < 0. 01) compared with seated body position. Post-exercise ln LF was significantly lower in the supine position with elevated legs than in the seated body position (p < 0.05). No significant difference was found among the three different body positions for post-exercise ln HF (p > 0.05). Post-exercise time domain measures of HRV (RRNN, SDNN, RMSSD) were significantly lower compared with baseline values (p < 0.01) regardless body position. Post-exercise ln LF and ln HF in all three positions remained significantly reduced during recovery compared to baseline values (p < 0.01). The present study suggests that 15 minutes following short-term submaximal exercise most of the time and frequency domain HRV measures have not returned to pre-exercise values. Modifications in autonomic cardiac regulation induced by body posture present at rest remained after exercise, but the post-exercise differences among the three positions did not resemble the ones established at rest. |
Key words:
Heart rate variability, recovery, exercise
|
Key
Points
- Whether different body positions may enhance post-exercise recovery of autonomic regulation remains unclear.
- The absence of restoration of HRV measures after 15 minutes of recovery favor the existence of modifying effects of exercise on mechanisms underlying heart regulation.
- On the basis of discrepancies in HRV measures in different body positions pre- and post-exercise we argue that the pace of recovery of cardiac autonomic regulation is dependent on body posture.
|
The autonomic nervous system (ANS) exerts a fine degree of control over the cardiovascular system (Tulppo and Huikuri, 2004). The evaluation of heart rate variability (HRV) at rest, during and after exercise is an innovative approach to study the different underlying physiological control mechanisms of the body reacting to physical activity (Sandercock and Brodie, 2006; Nunan et al., 2009; Torres et al., 2008). Acute effects of a single exercise bout on HRV revealed an initial decrease in HRV and vagal-related indices (e.g. standard deviation of normal-to-normal intervals, the root mean square of successive differences, high-frequency of HRV spectral power) after exercise (James et al., 2002; Javorka et al., 2002). Attempts to examine the kinetics of vagal-restoration were taken focusing on intensity of exercise (Gladwell et al., 2010; Neiwiadomski et al., 2007; Parekh and Lee, 2005; Terziotti et al., 2001), type of exercise (Heffernan et al., 2006; Mourot et al., 2004; Yamamoto et al., 2001), exercise duration (Seiler et al., 2007), recovery duration (Pober et al., 2004), and cardiorespiratory fitness (Buchheit and Gindre, 2006). Even though there is a great body of information on HRV recovery after exercise it is difficult to foresee with certainty the exact time frame for full autonomic recovery. HRV measures can reach pre- exercise values within 5 minutes when the exercise intensity is low and duration is short (Seiler et al., 2007; Terziotti et al., 2001). On the other hand high intensity exercise induces prolonged vagal reactivation and HRV recovery with progressive increase of high- and low-frequency HRV power indices that still might not reach resting values after 10 minutes (Arai et al., 1989; Pober et al., 2004; Takahashi et al., 2000), 15 minutes (Gladwell at al., 2010; Mourot et al., 2004; Pober et al., 2004; Terziotti et al., 2001), 30 minutes (Javorka et al., 2002) or even one hour (Furlan et al., 1993; James et al., 2002; Mourot et al., 2004; Terziotti et al., 2001). The time course of HRV recovery is definitely a function of exercise intensity and modality (Gladwell et al., 2010; Kaikkonen et al., 2008) but other physiological conditions (e.g. body position) might have a modifying influence on post- exercise ANS activity as well. Few attempts have been made to ascertain how changes in posture influence the dynamics of cardiovascular responses during recovery. Takahashi et al. utilised continuous non-invasive measurements of HRV in the upright and supine position and their results indicated that the restoration of post-exercise vagal activity was retarded, regardless of body position (Takahashi et al., 2000). Buchheit et al., 2009 investigated four recovery positions with emphasis on early parasympathetic reactivation (during the first 5 minutes of exercise) and found that the recovery of conventional HRV indices was moderately affected by body position. To our knowledge no articles have yet been published investigating the post-exercise autonomic activity of participants laying in the supine position with elevated legs for a 15 minute recovery period. In this position increased central blood volume via arterial baroreceptors imposes a greater vagal activation than in the supine position and reduces heart rate and cardiac output (Rowell, 1993). Greater vagal modification might lead to a faster recovery of cardiac autonomic control. We hypothesize that the mechanisms that exert postural effects on HRV in different body positions have alternate pace of recovery leading to differences in the recovery of HRV indices. The purpose of the present study is to reveal if there is a difference in the recovery of HRV measures depending on body position. Autonomic recovery as a function of body posture may have significant value in the prescription of safe and effective recovery after submaximal exercise. Therefore, the goal of this study was to assess the effect of three different body positions on HRV measures following short- term submaximal exercise and to investigate the differences in HRV measures before and after submaximal exercise.
ParticipantsThirty healthy young male students (mean±SD, age 20.5 ± 0.6 yrs, stature 181.1 ± 6.1cm, body mass 81.4 ± 9.2 kg) gave written informed consent to take part in the study. The participants were not engaged in any organized physical activity for the last six months before the start of the investigation. All subjects were in self- reported good health, without medications and had no medical histories from cardiovascular diseases. They also underwent a general physical examination to exclude any acute diseases and ailments of the cardiorespiratory and locomotor system. To assess individual differences in peak heart rate (HRpeak) 30 second all-out Wingate test was used and peak heart rate was measured as 183.1 ± 3.1 beats per minute at peak power of 768.6 ± 84.5 W. In highly cooperative participants peak HR during Wingate test might reach up to 94% of maximal HR obtained in aerobic cycling tasks (Hebestreit et al., 1993). All procedures conformed to the Declaration of Helsinki and were approved by the local ethics committee.
Instrumentation and data acquisition Measurements were undertaken in a quiet room, air temperature ranging from 22 to 24 °C, between 9 and 12h. Subjects were instructed to perform no strenuous exercise and have a solid night’s rest the day before the testing. After 10 minutes of accommodation, digital ECG (VNS-Spektr, Neurosoft, Ivanovo, Russian Federation) was recorded at rest, during exercise and recovery. A sampling rate of 1000 Hz was chosen and recordings were transferred to a PC via a USB interface. The epochs gained from the V5 lead were saved in a computer for further analysis. All R-R intervals were edited by visual inspection to exclude all the undesirable or ectopic beats. They were deleted with the post extra systolic beat and replaced automatically with interpolated adjacent R-R interval values.
Study design Participants were asked to visit the exercise laboratory on four different occasions (trials). On the first day peak power and peak HR were estimated by the Wingate anaerobic test and during the subsequent three visits the HRV measurements were performed. Subjects were randomly allocated to one of three equally sized groups relating to the order in which each group performed HRV measurements in different body position (seated, supine, supine with elevated legs). In the seated position, the participants’ feet were placed on a platform in front of the pedals while both legs were flexed at the knee at about 90°. This way most of the body weight was concentrated on the seat. The arms were placed over the thighs. In this position, participants reported that they felt comfortable and relaxed. In the supine body position, participants were asked to lie facing upwards, flat on the bed which was located next to the cycle ergometer. And finally, in the supine position with elevated legs the lower legs were placed horizontally on a pillowed platform placed at the end of the bed while the thighs were vertical to the upper body. In this position both legs were flexed in the knee at approximately 90°. The ECG recording was performed for five minutes at rest in seated, supine or supine with elevated legs body position on different days. Following resting (baseline) measurements, participants underwent a five minute submaximal cycling (Wattbike cycle ergometer, Wattbike Ltd, Nottingham, UK) with an intensity of 80% of individual peak HR values. A high submaximal load was used to evoke a substantial cardiac autonomic response. Heart rate values observed at the end of exercise (for each experimental protocol followed by recovery in the adequate body position - seated, supine and supine with elevated legs) were 142.9 ± 2.5 beats per minute (at 129.8 ± 7.9 W), 141.8 ± 1.9 beats per minute (at 130.5 ± 7.2 W) and 143.0 ± 2.3 beats per minute (at 131.3 ± 8.0 W), respectively. Upon cessation of exercise, the ECG recording was continued during the 15-minute recovery period in the same body position as before exercise (Figure 1). Following the exercise, subjects managed to move into the required supine body position in less than 5 seconds.
HRV analysis The mean RR normal-to- normal intervals (RRNN), the standard deviation of normal-to-normal intervals (SDNN), the root mean square of successive differences (RMSSD) were obtained as time domain measures. Frequency domain measures of HRV were derived by fast Fourier transformation: they were low-frequency (LF; 0.04-0.15 Hz) and high-frequency (HF; 0.15-0.40 Hz) spectral power (Task Force, 1996). Measures of TP and VLF were not included in further analysis as their interpretation is questionable when obtained from short 5-minute recordings (Sandercock et al., 2004). Temporal and spectral measures of HRV computed from short term recordings were reported to be reproducible and reliable (Carrasco et al., 2003, Sandercock et al., 2004, Sandercock et al., 2005, Nunan et al., 2009).
Data analysisThe distribution of each variable was examined with the Lilliefors normality test. Since the absolute HF and LF power values were skewed, power densities were transformed by taking their natural logarithms to allow parametric statistical comparisons that assume normal distributions (Sandercock et al., 2007). For testing differences paired t test was used (for baseline vs. post-exercise one-tailed, otherwise two-tailed). Working hypothesis was that two independent samples come from normal distributions with equal means. The test used was the Behrens- Fisher problem, as there were unknown and unequal variances. All statistical analyses were carried out using MatLab 6 software (The MathWorks Inc., Natick, MA, USA). Statistical significance was indicated if p < 0.05. All data are presented as means ± SD unless otherwise indicated.
Time and frequency domains of HRV measures with different body positions before and after exercise are presented in Table 1. At baseline there were higher values of RRNN (+17.6%), SDNN (+27.2%) and RMSSD (+86.3%) in the supine and of RRNN (+15.2%) and RMSSD (+50.6%) in the supine position with elevated legs (p < 0.01) compared with seated body position. No significant difference was found between the two supine positions regarding any time and frequency domain HRV measures. At baseline, the ln LF component of HRV was significantly lower in supine position with elevated legs the than in the seated position (- 5.6%) (p < 0.05). In contrast, the ln HF component of HRV was significantly higher in supine (+12.5%) (p < 0.01) and supine with elevated legs body position (+7.2%) (p < 0.05) than in the seated position. No significant difference was found between the two supine positions during the recovery period for any measured time or frequency domain variable of HRV (p > 0.05). Post-exercise values of time domain HRV variables were higher in the supine position: RRNN (+14.9%), RMSSD (+36.6%) and in the supine position with elevated legs: RRNN (+17.3%), RMSSD (+38.6%) (p < 0.01) compared with the seated body position. Post-exercise ln LF was significantly lower in the supine position with elevated legs (-7.5%) than in the seated body position (p < 0.05). No significant difference was found among the three different body positions for post-exercise ln HF (p > 0.05). Relative changes of time and frequency domain HRV parameters pre- and post-exercise are presented in Figure 2. Post-exercise time domain measures of HRV (RRNN, SDNN, RMSSD) were significantly lower compared with baseline values (p < 0.01) regardless body position. Post- exercise ln LF and ln HF in all three positions remained significantly reduced during recovery compared to baseline values (p < 0.01).
In the present study we evaluated the effects of different body positions on HRV measures at rest (baseline) and after short-term submaximal exercise. Most of the measured HRV variables did not return to the baseline values 15 minutes following exercise. Most importantly, the present study suggests that body position may influence HRV measures not only at baseline but also following submaximal exercise. In this investigation, baseline values of all time domain HRV parameters (RRNN, SDNN, RMSSD) were significantly higher in the two supine positions compared with the seated position, suggesting that parasympathetic activity is related to postural effects. Similarly ln HF was significantly greater in the supine position (with and without elevated legs) than in the upright position on the cycle ergometer. The review of Perini and Veicsteinas states that high frequency fluctuations during supine and sitting seem to be larger than during standing (Perini and Veicsteinas, 2003). The ratio of low- to high-frequency fluctuation during standing was significantly higher than during supine and sitting (Perini and Veicsteinas, 2003). This was linked to the parasympathetic predominance during supine and sitting, and a shift to sympathetic dominance while standing (Perini and Veicsteinas, 2003). The distribution of blood volume in the body in the upright position is affected profoundly by gravitational force. Adjustment of arterial blood pressure to compensate for postural change has been found to be achieved through a number of reflex mechanisms (e.g. arterial baroreflexes, cardiopulmonary baroreflexes) which may lead to a greater engagement of the sympathetic nervous system (Rowel, 1993). Pre-exercise sympathetic nerve activity in the supine position with elevated legs, expressed through lower ln LF, found in the present study, was probably reflexly suppressed by arterial baroreceptors. These were loaded by greater blood volume in central veins translocated from the lower extremities, compared with seated upright or the other supine position. In the present study we wanted to obtain further evidence regarding the recovery of autonomic control after exercise. The rationale for choosing 80% of peak HR was that we sought to implement a high submaximal load in order to evoke substantial cardiac autonomic response. There are no “golden standards” for training load prescription but it has generally been measured with heart rate, oxygen uptake, blood lactate concentration and rating of perceived exertion (Kaikkonen et al., 2010). Although much of the variability in physiological responses to submaximal exercise may result because the contribution of aerobic and anaerobic systems differs considerably as a function of maximal HR and VO2max (Meyer et al., 1999, Hofmann et al., 2001), most of the articles dealing with post-exercise HRV recovery define workloads as a percentage of HRmax or VO2max, (Takahashi et al., 2000, Parekh et al., 2005, Kaikkonen et al., 2010) anaerobic threshold (Terziotti et al., 2001) or blood lactate level (Gladwell et al., 2010). We chose fixed intensities targeting percentage of individual peak HR values, but the untrained participants reached similar workloads throughout all three testing conditions forming homogenous groups for power values. In this study all the participants performed a 5 minute submaximal exercise followed by a recovery period of 15 minutes in different body positions. Terziotti et al. showed that after 15 minutes of recovery the autonomic control of the cardiovascular system was still altered, and the tone of the vagus did not fully recover (depressed HF power) after exercise at both 50 and 80% of the anaerobic threshold (Terziotti et al., 2001). Parekh and Lee examined cardiac autonomic modulation via HRV after exercise bouts at 50 and 80% of VO2 reserve. The HRV measures were examined in 5 minute periods of a 30 minute post- exercise supine recovery. They showed that vagal reactivation was delayed for a longer period after high intensity exercise bout (HF restored after 25 minutes) compared to low intensity (HF restored after 10 minutes) (Parekh and Lee, 2005). Gladwell et al. also demonstrated HF recovery in the supine position 15 minutes after moderate exercise intensity and 30 minutes after hard and severe intensities (Gladwell et al., 2010). Post-exercise delay in restoration of vagal activity was demonstrated through decreased time domain HRV parameters and the ln HF component of HRV, regardless of body positions. Although the time domain measures of HRV were significantly lower post exercise in all body positions compared with baseline values they remained higher in the supine positions compared with the upright seated position. This suggests that a full recovery of time domain HRV measures was not achieved after 15 minutes but the postural effects of body position remained evident, i.e. the discrepancies in HRV among the three body positions post-exercise were not of the same extent as at rest. Thus, when considering the effects of exercise on HRV recovery the extent of recovery should also be considered. In agreement with previously cited studies (James et al., 2002, Terziotti et al., 2001, Mourot et al., 2004), the high- and low-frequency fluctuations in HRV remained reduced compared with baseline values following exercise. The ln HF fluctuations have not recovered after 15 minutes regardless of body position but the between position difference that existed at rest (between the seated and supine position about 12.5% and the seated and supine position with elevated legs about 7.2%) disappeared post-exercise. The ln LF power of HRV also remained significantly reduced compared with baseline values for all positions. Takahashi et al. also demonstrated lower amplitudes of HF compared with those at rest before exercise in the upright and supine body positions indicated that the restoration of activity in the post-exercise vagal activity was delayed, regardless of body position (Takahashi et al., 2000). Lower HRV values following exercise might be explained through inhibiting effects of sympathetic blood hormones on vagal modulation (Miyamoto et al., 2003). Metaboreceptors with sympathetic afferents might also be activated by accumulated waste product during exercise (O`Leary, 1993) proposing an alternative mechanism for sympathetic predominance. Elimination of these influences from the blood is not affected by body posture abolishing between position differences in HRV recovery. Whether the differences in post-exercise HRV measures in relation to body position are merely a result of the influences present in baseline conditions is still unclear. The absence of restoration of HRV measures after 15 minutes of recovery favor the existence of modifying effects of exercise on mechanisms underlying heart regulation (i.e. baroreflex or metaboreflex functions). On the basis of discrepancies in HRV measures in different body positions pre- and post-exercise we argue that the pace of recovery of cardiac autonomic regulation is dependent on body posture. After exercise cessation, lower preload in the upright position and the absence of central command mechanisms cause a drop in cardiac output and arterial blood pressure that is restored reflexly through increased sympathetic activity (Bradley and Davis, 2003, Westerhof et al., 2006). Cardiac sympathetic nerve activity decreases and vagus nerve activity increases when the body position changes from upright position to supine (Rowel, 1993). If a similar change in activity of the two components of the ANS occurs during the post-exercise period, a faster decrease in HR would be induced in the supine position via predominance of vagus nerve activity, compared with the upright position (Takahashi et al., 2000). Similar immediate responses would be evident in other aspects of cardiac autonomic activity as well. The baroreceptors involved in this reflex regulation are effective only at buffering short term changes, so major perturbations in ANS activity would be evident immediately after exercise cessation (Rowell, 1993). Further changes in HRV measures might not be explained merely by this reflex mechanism, but the weakening of baroreflex influence on post-exercise ANS activity after 15 minutes of recovery might explain the absence of significant difference between the two supine positions. Whether the redistribution of blood in the supine position with elevated legs is sufficient enough to evoke greater perturbations in the cardiac autonomic regulation than in a simple supine position is not proved conclusively.
The present study suggests that 15 minutes following short- term submaximal exercise most of the time and frequency domain HRV measures have not returned to pre-exercise values regardless of body position. Modifications in autonomic cardiac regulation induced by body posture present at rest remained after exercise, but the post-exercise differences among the three positions did not resemble the ones established at rest. The question remains if there is any favorable body position that will accelerate the recovery of cardiac autonomic regulation.
ACKNOWLEDGEMENTS |
This study was funded with a research grant provided by the Provincial Secretariat for Science and Technological Development, Autonomous Province of Vojvodina, Serbia; Contract grants number: 114-451-00717/2009. |
|
AUTHOR BIOGRAPHY |
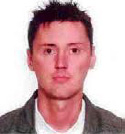 |
Otto F. Barak |
Employment: PhD student , Medical School, University of Novi Sad, Novi Sad, Serbia. |
Degree: MSc, MD. |
Research interests: Exercise physiology, cardiovascular health, cardiac autonomic regulation, physical activity and recovery. |
E-mail: ottompotom@uns.ac.rs |
|
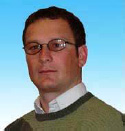 |
Djordje G. Jakovljevic |
Employment: Researcher, Newcastle Magnetic Resonance Centre, Muscle Performance and Exercise Training Laboratory, Institute for Ageing and Health, Newcastle University, UK. |
Degree: PhD. |
Research interests: Exercise physiology in health and disease with particular focus on overall cardiac functions. |
E-mail: d.jakovljevic@newcastle.ac.uk |
|
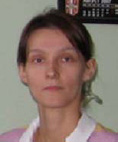 |
Jelena Z. Popadic Gacesa |
Employment: Assistant professor of Physiology, Medical School, University of Novi Sad, Novi Sad, Serbia. |
Degree: PhD, MD. |
Research interests: Exercise physiology, body composition, muscle adaptation to exercise. |
E-mail: jpopadic@uns.ac.rs |
|
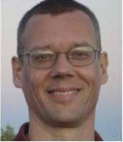 |
Zoran B. Ovcin |
Employment: Assistant of Mathematics, Faculty of Technical Sciences, University of Novi Sad, Novi Sad, Serbia. |
Degree: MSc. |
Research interests: Mathematics, numerical analysis. |
E-mail: zovcin@uns.ac.rs |
|
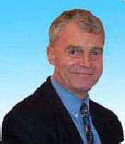 |
David A. Brodie |
Employment: Professor of Cardiovascular Health, Head of Research, Research Centre for Society and Health, Buckinghamshire New University, UK. |
Degree: BPhD, MD. |
Research interests: Cardiovascular health as applied to healthy and unhealthy populations, cardiac rehabilitation. |
E-mail: david.brodie@bucks.ac.uk |
|
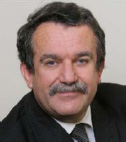 |
Nikola G. Grujic |
Employment: Dean of Faculty, Professor of Physiology and Sports Medicine, Medical School, University of Novi Sad, Novi Sad, Serbia. |
Degree: PhD, MD. |
Research interests: Exercise physiology, ergometry, rowing, sudden cardiac death. |
E-mail: grunik@eunet |
|
|
|
REFERENCES |
 Arai Y., Saul J.P., Albrecht P., Hartley L.H., Lilly L.S., Cohen R.J., Colucci W.S. (1989) Modulation of cardiac autonomic activity during and immediately after exercise. American Journal of Physiology 256, H132-H141.
|
 Bradley J.G., Davi K.A. (2003) Orthostatic hypotension. American Family Physician 68, 2393-2398.
|
 Buchheit M., Al Haddad H., Laursen P.B., Ahmaidi S. (2009) Effects of body posture on parasympathetic reactivation in men. Experimental Physiology 94, 795-804.
|
 Buchheit M., Gindre C. (2006) Cardiac parasympathetic regulation: respective associations with cardiorespiratory fitness and training load. American Journal of Physiology: Heart and Circulatory Physiology 291, H451-H458.
|
 Carrasco S., Gonzalez R., Gaitan M.J., Yanez O. (2003) Reproducibility of heart rate variability from short-term recordings during five manoeuvres in normal subjects. Journal of Medical Engineering and Technology 27, 241-248.
|
 Furlan R., Piazza S., Dell'Orto S., Gentile E., Cerutti S., Pagani M., Malliani A. (1993) Early and late effects of exercise and athletic training on neural mechanisms controlling heart rate. Cardiovascular Research 27, 482-488.
|
 Gladwell V.F., Sandercock G.R.H., Birch S.L. (2010) Cardiac vagal activity following trhree intensities of exercise in humans. Clinical Physiology and Functional Imaging 30, 17-22.
|
 Hebestreit H., Mimura K-I., Bar-Or O. (1993) Recovery of muscle power after high-intensity short-term exercise: comparing boys and men. Journal of Applied Physiology 74, 2875-2880.
|
 Heffernan K.S., Kelly E.E., Collier S.R., Fernhall B. (2006) Cardiac autonomic modulation during recovery from acute endurance versus resistance exercise. European Journal of Cardiovascular Prevention and Rehabilitation 13, 80-86.
|
 Hoffmann P., Von Duvillard S.P., Seibert F-J., Pokan R., Wonisch M., Lemura L., Schwaberger G. (2001) HRmax target heart rate is dependent on heart rate performance curve deflection. Medicine and Science in Sports and Exercise 33, 1726-1731.
|
 James D.V.B., Barnes A.J., Lopes P., Wood D.M. (2002) Heart rate variability: response following a single bout of interval training. International Journal of Sports Medicine 23, 247-251.
|
 Javorka M., Zila I., Balharek T., Javorka K. (2002) Heart rate recovery after exercise: relations to heart rate variability and complexity. Brazilian Journal of Medical and Biological Research 35, 991-1000.
|
 Kaikkonen P., Hynynen E., Mann T., Rusko H., Nummela A. (2010) Can HRV be used to evaluate training load in constant load exercises?. European Journal of Applied Physiology 108, 435-442.
|
 Kaikkonen P., Rusko H., Martinmaki K. (2008) Post-exercise heart rate variability of endurance athletes after different high-intensity exercise interventions. Scandinavian Journal of Medicine and Science in Sports 118, 511-519.
|
 Meyer T., Gabriel H.H., Kindermann W. (1999) Is determination of exercise intensities as percentages of VO2max or HRmax adequate?. Medicine and Science in Sports and Exercise 31, 1342-1345.
|
 Miyamoto T., Kawada T., Takaki H., Inagaki M., Yanagiya Y., Jin Y., Sugimachi M., Sunagawa K. (2003) High plasma norepinephrine attenuates the dynamic heart rate response to vagal stimulation. American Journal of Physiology: Heart and Circulatory Physiology 284, H2412-H2418.
|
 Mourot L., Bouhaddi M., Tordi N., Rouillon J.D., Regnard J. (2004) Short- and long-term effects of a single bout of exercise on heart rate variability: comparison between constant and interval training exercises. European Journal of Applied Physiology 92, 508-517.
|
 Neiwiadomski W., Gasiorowska A., Krauss B., Mroz A., Cybulski G. (2007) Supression of heart rate variability after supramaximal exertion. Clinical Physiology and Functional Imaging 27, 309-319.
|
 Nunan D., Donovan G., Jakovljevic D.G., Hodges L.D., Sandercock G.R., Brodie D.A. (2009) Validity and reliability of short-term heart-rate variability from the Polar S810. Medicine and Science in Sports and Exercise 41, 243-50.
|
 O'Leary D.S. (1993) Autonomic mechanisms of muscle metaboreflex control of heart rate. Journal of Applied Physiology 74, 1748-1754.
|
 Parekh A., Lee C.M. (2005) Heart rate variability after isocaloric exercise bouts of different intensities. Medicine and Science in Sports and Exercise 37, 599-605.
|
 Perini R., Veicsteinas A. (2003) Heart rate variability and autonomic activity at rest and during exercise in various physiological conditions. European Journal of Applied Physiology 90, 317-325.
|
 Pober D.M., Braun B., Freedbon P.S. (2004) Effects of a single bout of exercise on resting heart rate variability. Medicine and Science in Sports and Exercise 36, 1140-1148.
|
 Rowell L.B, Rowell L.B (1993) Human cardiovascular control. Reflex control during orthostasis. New York. Oxford University Press.
|
 Sandercock G.R.H. (2007) Normative values, reliability and sample size estimates in heart rate variability. Clinical Science 113, 129-130.
|
 Sandercock G.R.H., Brodie D.A. (2006) The use of heart rate variability measures to assess autonomic control during exercise. Scandinavian Journal of Medicine and Science in Sports 116, 302-313.
|
 Sandrecock G.R.H., Bromley P., Brodie D.A. (2004) Reliability of three commercially available heart rate variability instruments using short-term (5-min) recordings. Clinical Physiology and Functional Imaging 24, 359-367.
|
 Sandrecock G.R.H., Bromley P., Brodie D.A. (2005) The reliability of short-term measurements of heart rate variability. International Journal of Cardiology 103, 238-247.
|
 Seiler S., Haugen O., Kuffel E. (2007) Autonomic recovery after exercise in trained athletes: inensity and duration effects. Medicine and Science in Sports and Exercise 39, 1366-1373.
|
 Takahashi T., Okada A., Saitoh T., Hayano J., Miyamoto Y. (2000) Difference in human cardiovascular response between upright and supine recovery from upright cycle exerise. European Journal of Applied Physiology 881, 233-239.
|
 Task Force of the European Society of Cardiology the North American Society of Pacing Electrophysiology. (1996) Heart Rate Variability. Standards of measurement, physiological interpretation and clinical use. European Heart Journal 17, 354-381.
|
 Terziotti P., Schena F., Gulli G., Cevese A. (2001) Post-exercise recovery of autonomic cardiovascular control: a study by spectrum and cross-spectrum analysis in humans. European Journal of Applied Physiology 84, 187-194.
|
 Torres B.C., Lopez C.L., Orellana J.N. (2008) Analysis of heart rate variability at rest and during aerobic exercise: a study in healthy people and cardiac patients. British Journal of Sports Medicine 42, 715-720.
|
 Tulppo M.P., Huikuri H.V. (2004) Origin and significance of heart rate variability. Journal of the American College of Cardiology 43, 2278-80.
|
 Westerhof B.E., Gisolf J., Karemaker J.M., Wesseling K.H., Secher N.H., van Lieshout J.J. (2006) Time course of baroreflex sensitivity during postural stress. American Journal of Physiology: Heart and Circulatory Physiology 291, H2864-H2874.
|
 Yamamoto K., Miyachi M., Saitoh T., Yoshioka A., Onodera S. (2001) Effects of endurance training on resting and post-exercise cardiac autonomic control. Medicine and Science in Sports and Exercise 33, 1496-1502.
|
|
|
|
|
|
|