|
|
|
ABSTRACT |
The aim of this study was to determine the validity and reliability of a microelectromechanicals (MEMs) based system (Keimove™) in measuring flight time and takeoff velocity during a counter-movement jump (CMJ). As criterion reference, data of a high- speed camera (HSC) and a force-platform (FP) synchronized with a linear position transducer (LPT) was used. Thirty professional soccer players completely familiarized with the CMJ technique performed three CMJs. The second and third trials were used for further analysis. The Keimove™ system, the HSC and the FP synchronized with the LPT (FP+LPT) simultaneously measured the CMJ performance. During each repetition, the Keimove™ system registered flight time and velocity at takeoff. At the same time and as criterion reference, both the HSC and the FP recorded the flight time while the LPT+FP registered the velocity at takeoff. Pearson correlation coefficients for the flight time were high (r = 0.99; p < 0.001) when Keimove™ system was compared with the HSC or the FP+LPT, respectively. For the velocity at takeoff variable, the Pearson r between the Keimove™ system and the FP+LPT was lower although significant at the 0.05 level. No significant differences in mean values were observed for flight times and velocity at takeoff between the three devices. Intraclass correlations and coefficients of variation between trials were similar and ranged between 0.92-0.97 and 2.1-7.4, respectively. In conclusion, the Keimove™ system represents a valid and reliable instrument to measure velocity at takeoff and flight time during CMJ testing. Thus, this MEMs-based system will offer a portable, cost-effective tool for the assessment CMJ performance. |
Key words:
Counter-movement jump, microelectromechanical systems, testing
|
Key
Points
- The Keimove™ system is composed of specific software and a wireless MEMs-based device designed to be attached at the lumbar region of the athlete.
- The Keimove™ system is a mechanically valid and reliable instrument in measuring flight time and velocity at takeoff during CMJ.
- The validity of the Keimove™ system was determined by comparing its mean flight time and velocity at takeoff with data obtained simultaneously with both a high-speed camera and a force platform synchronized with a linear position transducer.
|
Vertical jumping is an essential motor skill in many sports (Ziv and Lidor, 2010). Although sport performance depends on many factors, the ability of an athlete to reach a point as high above the ground as possible may often determine the difference between success and failure. Consequently, in the last decades much research has been focused on biomechanical analysis of human vertical jumping ability (Bobbert and Van Soest, 2001; Reiser et al., 2006) and determining which training strategies are more suitable to increase vertical jumping performance (Baker, 1996; Schmidtbleicher, 1992; Sleivert and Taingahue, 2004; Wilson et al., 1997). Many methods and tools have been used to assess vertical jump performance (i.e., Aragon- Vargas, 2000; Bosco et al., 1983; García-López et al., 2005; Isaacs, 1998; Innocenti et al., 2006; Quagliarella et al., 2010). These include force platforms (i.e., Dowling and Vamos, 2003; Hatze, 1998), optical cells/contact mats (i.e., Bosco et al., 1983; García-López et al., 2005), jump and reach test devices (i.e. Isaacs, 1998) and the use of video technique (Hatze, 1998). It has been indicated that the video technique, which measures the displacement of the center of gravity of the body from the standing position to the highest vertical displacement, must be considered as the criterion reference or the gold standard method for vertical jumping measurement (Klavora, 2000). However, this method is not frequently used because it requires expensive motion analysis equipment that is difficult to calibrate and transport. In addition, jump performance data are obtained after a posterior analysis and require trained individuals to accurately operate. The use of force platforms to measure vertical jump performance is another well-validated and extensively used method in scientific literature. Similarly to the visual analysis, this assessment must take place in the laboratory and not in the field because force platforms are very sensitive to extraneous vibrations and therefore must be mounted as specified by the manufacturer’s instructions to preserve the integrity of the signal. Because of these reasons, several researchers (Cronin et al., 2004; Leard et al., 2007; Innocenti et al., 2006; Quagliarella et al., 2010) have validated different portable and cost-effective equipment (e.g., optical cell/contact mats or jump and reach devices) to measure vertical jump performance taking as a criterion reference both video technique and/or force platform methods. For example, Cronin et al., 2004 showed that the calculations derived from a linear position transducer (LPT) highly correlated with those obtained simultaneously with a force platform (FP). In the last years, many studies (i.e., Coventry et al., 2006; Innocenti et al., 2006; Mayagoitia et al., 2002; Quagliarella et al., 2010; Sleivert and Taingahue, 2004) have used microelectromechanical systems (MEMs) devices - normally accelerometers and gyroscopes - fixed at different parts of the body to obtain several kinematic values. Most of these studies have been performed in the clinical biomechanics context to measure bone segment acceleration (i.e., Hopper et al., 2002) and analyze mechanical shock during landing (i.e., Coventry et al., 2006). In the case of vertical jump, four studies (Casartelli et al., 2010; Innocenti et al., 2006; Quagliarella et al., 2010; Sleivert and Taingahue, 2004) have been reviewed in which body mounted MEMs were used to determine an athlete’s vertical jump performance. For example, Quagliarella and co-workers tried to compare flight time data obtained by a MEMs-based system (two bi-axial accelerometers fixed at each subject’s ankle) with flight time from a force platform, showing a high correlation between both devices (Quagliarella et al., 2010). However, more information is still needed to confirm the validity and reliability of these body- mounted kinematic sensors to determine vertical jump performance. In this sense, only one of these studies used a tri-axial accelerometer (Casartelli et al., 2010). The rest of the studies used MEM devices composed exclusively of mono/bi-axial accelerometers connected to a body-wearable data logger (non-telemetric systems). All the reviewed studies fixed the MEMs device at different positions such as the Smith machine bar (Sleivert and Taingahue, 2004), ankle (Quagliarella et al., 2010) or hip (Casartelli et al., 2010). Curiously, none of these studies positioned the MEMs device on the low back where an instantaneous acceleration of a point reasonably close to the centerof mass (Hasan, 1996; Winter, 1990) can be obtained. Finally and most importantly, none of the reviewed studies include other important MEMs to human movement analysis such as gyroscopes. For the present study, a new commercial MEMs- based system was used that is light ( 20 gr), telemetric, composed of tri-axial MEMs synchronized and designed to be fixed at the nearest position of the human body center of mass. Thus, the purpose of the present study was to determine whether the performance variables selected (flight time and takeoff velocity) of a counter-movement jump (CMJ) as measured by this MEMs based-system (Keimove™) were reliable and similar to the information gathered simultaneously on a high-speed camera (HSC) and a FP synchronized with a LPT (criterion reference). If proven valid and reliable, this MEMs-based system will offer a portable, cost-effective tool for the assessment of CMJ performance.
Experimental designThe reliability and validity of a wireless MEMs-based system (Keimove™) to measure CMJ performance was determined by comparing flight time and velocity at takeoff measurements with data obtained simultaneously with a HSC and a FP synchronized with a LPT.
ParticipantsThirty male subjects volunteered to participate in this study. Their mean (SD) age, height and body mass were 18 (2.8) years, 179.3 (7.3) cm and 75.0 (6.9) kg, respectively. All subjects were professional soccer players who trained on a daily basis (2-3 h per day). These players were completely familiarized with the CMJ technique. All the players were informed of the procedures and the purpose of the study and their written informed consent was obtained. The Human Subject Ethics Committee of the Pablo de Olavide University approved all procedures undertaken in this study.
EquipmentThe Keimove™ system (Vincid Research, S.L., Granada, Spain) is composed of specific software and a wireless MEMs-based device (Keimove™ measurement unit) designed to be attached at the lumbar region of the athlete (the nearest zone of the center of mass) with a specially designed belt (Figure 1). In the present study, special attention was paid to using the Keimove™ system in accordance with the instructions of the manufacturer. In this sense, the skin area located at L4-L5 vertebral levels was considered representative of body center of mass during upright stance (Hasan et al., 1996; Winter, 1990). The Keimove™ measurement unit (dimensions: 4.5 x 4 x 1.3 cm; weight: 19 g) is composed principally of several 3D-synchronized MEMs such as a 3D inertial accelerometer (± 8 g of full range), a 3D gyroscope, both of which record at a sample frequency of 1 KHz, and a magnetometer. The Keimove™ measurement unit data were sent to a laptop computer via Bluetooth®. The Keimove™ software permits visualizing live 3D linear acceleration-, 3D linear velocity- and 3D angular velocity-time curves during the subject's movement. For the purposes of the present study, the Keimove™ software automatically calculated flight time and linear velocity of body center of mass at takeoff moment from each CMJ. Flight time was determined as the time interval in which the vertical acceleration was found to be equal to or lower than gravitational acceleration. Takeoff was determined as the instant before the flight phase. Velocity at takeoff was obtained from the integration of the acceleration recordings. Indeed, considering that the Keimove™ measurement unit was manually positioned on the participant's back and that during the CMJ the trunk deviates from the vertical direction mainly due to bending of the participant’s sagittal plane, the sensor’s acceleration (measured with respect to the moving Keimove™ measurement unit case) was expressed with respect to a fixed global system of reference, which was made to coincide with the participant’s sagittal plane in a standing posture. This was done by fusing the 3D accelerometer and gyroscope data. To measure velocity at takeoff as the criterion reference measurement, a FP (IsoNet, JLML, Madrid, Spain) synchronized with a LPT (Isocontrol, JLML, Madrid, Spain) was used (Figure 2). The LPT was countersunk into a custom-made base as it has previously been described (Cronin et al., 2004) (Figure 1B). The wire from the transducer was attached to a waist harness on the subject and measured vertical displacement relative to the base with an accuracy of 0.1 cm. The LPT recorded the position and direction of the wire with an accuracy of 0.2 mm and time events with an accuracy of 1 ms. The transducer and base were placed upon the force platform. This arrangement allowed simultaneous data collection from the LPT and the FP during jumping. The FP selected used a sampling rate of 1 KHz. A custom-designed Labview (National Instruments, Austin, TX) FP and LPT analysis software was used to calculate the variables of interest. From the LPT data (position-time curve), the velocity-time curve was measured at a point near to the body center of mass during the CMJ. From the FP data, takeoff and landing moments were determined on the LPT velocity-time curve. To measure flight time as criterion reference measurement the above indicated force platform and two high-speed cameras (HSC) (EXFH20, Casio, Japan) were used (Figure1 B). The HSCs allowed to film at 1000 fps and were placed frontally and laterally (50 cm separated) at feet height. Time elapsed between take off and landing photograms was registered for further analysis (Figure 2).
ProceduresThe Keimove™ system, the HSCs and the FP synchronized with the LPT (FP+LPT) measured all the CMJs simultaneously. During each repetition, the Keimove™ system registered flight time and velocity at takeoff (Figure 3). At the same time and as criterion reference, both the HSCs and the FP obtained the flight time while the LPT+FP registered the velocity at takeoff (Figure 2). During testing, the players participated in their regular conditioning, sport and strength training routines. Adequate rest of 24 h was allowed between all training sessions and testing. Each player was tested separately. Once in the laboratory, each subject performed a warm-up that included low intensity running, dynamic stretching and 3 sets of 4 submaximal CMJs with 2-3 min of recovery between sets. Although these subjects were familiarized with CMJ testing, during the last part of the warm-up subjects were again instructed regarding the performance of CMJ. Using a preparatory countermovement, the subjects initiated the jump from an extended leg position, descended to 90° knee flexion, and immediately performed an explosive concentric action for maximal height. The subjects moved their arms freely during jumping. To film takeoff and landing moments with higher precision, the players jumped barefoot. Before the jump assessment, Keimove™ system and the LPT were attached by harness to each subject. As indicated by the manufacturer, the elastic belt that housed the Keimove™ was worn tightly and directly over the skin (under players' clothing) during CMJ testing. Three maximal CMJs were recorded with 1 min of rest. Trials 2 and 3 were used for analysis.
Statistical analysisValues are expressed as means ± SD from trials 2 and 3. Paired Student’s t-tests were carried out between variables (flight time and velocity at takeoff) to determine whether differences existed between means. The Pearson product moment correlation coefficient (r) was used to examine the validity of the flight time and the velocity at takeoff as measured by the different devices. Reliability was tested according to procedures outlined by Atkinson and Nevill, 1998. Reliability measurements were calculated from trials 2 and 3 in an effort to minimize measurement error, especially systematic bias. Trial 1 provided familiarization in addition to that provided in the warm-up. The “relative” reliability of the Keimove™ system was tested by assessing the intraclass correlation coefficient (ICC) between the flight time and the velocity at takeoff of the 2 trials measured with this device. These ICCs were compared with the same variables as calculated from the FP synchronized with the LPT (flight time and velocity at takeoff variables) and the HSC (only flight time variable). “Absolute” reliability was tested by the coefficient of variation (CV) for each single case, and then the mean CV was determined for the sample. A level of p < 0.05 was used for establishing statistical significance.
The mean values and SDs for flight time and velocity of takeoff variables (n = 30) and the Pearson product moment correlation coefficient for the jumps as assessed by the high-speed cameras, the force platform synchronized with the position transducer and the Keimove™ system can be observed in Table 1. Pearson correlation coefficients for the flight time were high (r = 0.98, p < 0.001) when Keimove™ system was compared with the HSC or the FP+LPT, respectively. For the velocity at takeoff variable, the Pearson r between the Keimove™ system and the FP+LPT was lower although significant at the 0.01 level. No significant differences in means were observed for flight times and velocity at takeoff between the three devices (Table 1). The trial-to-trial reliability of flight time and velocity at takeoff for the three (HSC, FP+LPT and Keimove™ system) and two (FP+LPT and Keimove™ system) devices, respectively, can be observed in Table 2. ICCs and CVs between devices were similar and ranged between 0.92-0.97 and 2.1-7.4, respectively (see Table 2).
In literature, numerous kinematic and kinetic measures have been used to determine CMJ performance (i.e., CMJ height or mean/peak ground reaction force). For the present study, we selected both the jump flight time because of it is extensive use in the related literature and the velocity of the body center of mass at takeoff suggested as the most descriptive variable of the vertical jumping performance (Reiser et al., 2006). Jump flight time and indirectly calculated jump height value measured by means of contact/laser mats may be considered the most used procedure in the literature to describe jump performance. However, it is well known that the takeoff and landing positions can affect the jump height recorded from a contact mat. Fall time can be extended by an athlete due to folding his legs and this situation may cause measurement error when jumping height is estimated (Aragon-Vargas, 2000; Musayev, 2006). To minimize this error, during vertical jump testing subjects are generally asked to take landing with their legs in complete extension (i.e., CMJ technique). For a maximum vertical jump, the most basic goal is to reach a point as high above the ground as possible. Taking into account that the body acts as a projectile once in the air, raising the position of the center of mass and the vertical velocity of the center of mass as high as possible at takeoff are the most important keys to success (Reiser et al., 2006). Indeed, the parabolic path of a projectile’s center of mass is determined by its position and velocity at the time it starts its flight; the center of mass cannot be altered once in the air until contact is made with the ground, another surface, object, or other player (Reiser et al., 2006). Thus, during a vertical jump in which the center of mass position is going to be the highest at the moment of takeoff, vertical velocity appears to be the main determinant performance variable. The main limitations of the present study were assuming that: (1) the Keimove™ measurement unit, placed on the low back at the level of L5, had the same kinematics than the LPT wire (attached 2 cm below) at the instant of takeoff; (2) the skin surface at L5 level was representative of the body center of mass during jumping. Both assumptions were necessary to append the ballistic motion to the kinematics of the Keimove™ /LPT wire prior to the flight phase. The primary finding of the present study was that the Keimove™ system is a mechanically valid and reliable instrument in measuring flight time and velocity at takeoff during CMJ. The accuracy of a new assessment tool is usually studied by comparing the new device with that of another (Rahmani et al., 2000; Thompson and Bemben, 1999; Wilson et al., 1997). In the present study, the validity of the Keimove™ system was determined by comparing its mean flight time and velocity at takeoff with data obtained simultaneously with both HSC and FP+LPT devices. If a high (r > 0.80) and statistically significant correlation coefficient is obtained between the two devices, the equipment is deemed to be sufficiently valid (Atkinson and Nevill, 1998). Pearson correlation coefficients greater than 0.90 were found for the variables measured (see Table 1) and consequently, the Keimove™ system measurements can be considered valid. The ICC represents the level of covariance from paired values in relation to the total variance (Atkinson and Nevill, 1998). It has been postulated that ICC values above 0.75 may be considered reliable and this index should be at least 0.90 for most clinical applications (Atkinson and Nevill, 1998). The ICCs for both flight time and velocity at takeoff measures assessed by the Keimove™ system meet this requirement. Moreover, for both measures in all the devices (Keimove™, HSC an FP+PT) the CVs were less than 5%. Several authors have arbitrarily chosen an analytical goal of the CV being 10% or below (Atkinson and Nevill, 1998; Hatze, 1998)). Thus, the high ICC and the low CVs and the absence of any statistical difference between trials indicated that the Keimove™ system data were reliable. Two recent studies (Casartelli et al., 2010; Quagliarella et al., 2010) have focused on the validity of inertial sensors based methods for the estimate of CMJ performance indicators with respect to criterion reference measurement instruments (i.e., “gold standard”) such as FP. Firstly, Quagliarella et al., 2010 compared flight time from a force platform with flight time from a MEMs-based system. In agreement with the present study, the flight times from the system proposed and the force platform during CMJ were highly correlated (> 0.95). However, in that study the MEMs system proposed was different to the one used in the present research Indeed, Quagliarella and co-workers used two bi-axial accelerometers mounted in each subject’s ankle and connected to a wearable system to proportionate flight time information after a posterior curve analysis (Quagliarella et al., 2010). In contrast, in the present study we used a wireless device (Keimove™) composed of tri-axial MEMs all of them synchronized that, by means of specific software, automatically calculate flight time - similarly to Quagliarella and co-workers’ device (Quagliarella et al., 2010) - and velocity at takeoff of the body center of mass during CMJ, both in real time. Secondly, Casartelli et al., 2010 compared flight height from a wearable accelerometric system (Myotest system) with flight height from validated photoelectric cells (Optojump). As well as Quagliarella et al., 2010, these authors used a MEMs based device clearly different to that used in the present study. Casartelli et al., 2010 used a non-telemetric device of larger dimensions (5.4 x 10.2 x 11.1 cm; weight: 58 g) characterized by containing one 3D inertial accelerometer and be fixed to the hip level. Accelerometric data were stored during the assessments and subsequently downloaded for jump height calculations using one specific software. This software automatically integrated the acceleration recordings to obtain vertical velocity, from which jump height was estimated by two calculation methods (method 1: using the flight time; method 2: using the highest vertical takeoff velocity). These authors conclude that the proposed device was a valid and reliable method for the assessment of vertical jump when flight time was recorded (method 1), despite significant overestimation compared to the criterion instrument used. In contrast, when velocity at takeoff variable it was used (method 2), this device was neither valid nor reliable. However, this study had two major limitations that make difficult the comparison with the results obtained by Quagliarella et al., 2010 and those observed in the present study. First, as criterion reference used a measurement instrument (photoelectric cells) non-considered criterion reference to quantify vertical jump height (Aragon-Vargas, 2000; Linthorne, 2001). Second, the device proposed did not include a 3D gyroscope synchronized with the accelerometer. As a result, real jump height data only be obtained if it can be ensured that the sensor is aligned with the absolute vertical direction and does not deviate from the latter during the execution of the CMJ (Innocenti et al., 2006; Veltink et al., 2003). Unfortunately, alignment problems may arise because of the manual positioning of the sensor on the body and due to rotations of the trunk during the CMJ. Because of this reason, the Keimove™’s acceleration was expressed with respect to a fixed global system of reference which was made to coincide with the participant’s sagittal plane during standing posture. To the best of our knowledge, this is the first validity study of a portable MEMs-based system designed to measure velocity at takeoff during vertical jumping. Future research validating its use during other jump modalities (drop jump or run-up vertical jumps) is clearly required.
The Keimove™ system represents a valid and reliable instrument to measure velocity at takeoff and flight time during vertical jump testing. Velocity at takeoff measure is often limited to laboratory-type assessment. This variable is considered the main descriptive variable of vertical jump (Musayev, 2006; Reiser et al., 2006) and its value is independent of body movement during flight time (i.e., landing body posture). Moreover, the Keimove™ system has the added advantage of being located near the body center of mass, which permits coaches to know exactly the mechanics registered at this point during the movement and the athletes to execute a vertical jump without any special restriction, such as normally generated by a FP or a contact mat. Thus, this MEMs-based system will offer a portable, cost-effective tool for the assessment CMJ performance.
ACKNOWLEDGEMENTS |
The Keimove™ system used for this investigation was donated to the Department of Human Performance at the University of Pablo de Olavide (Spain). The authors have no commercial or proprietary interest in this device |
|
AUTHOR BIOGRAPHY |
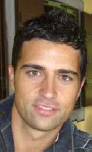 |
Bernardo Requena |
Employment: Professor at the Faculty of Sport – University of Pablo de Olavide, Spain. |
Degree: PhD |
Research interests: Biological basis for strength and power, mechanisms for adaptation in strength and power training and strength and power training for sports (especially soccer) |
E-mail: breqsan@upo.es |
|
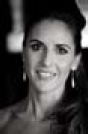 |
Inmaculada García |
Employment: Professor at the Faculty of Sport – University of Pablo de Olavide, Spain. |
Degree: PhD |
Research interests: Strength and conditioning. |
E-mail: igarcia@upo.es |
|
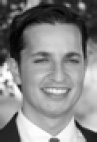 |
Francisco Requena |
Employment: Associate Professor of the Department of Orthopaedics and Traumatology, University of Granada, Spain. |
Degree: MD |
Research interests: Biomechanics of human locomotor system and Orthopaedics and Traumatology in sport context. |
E-mail: franciscorequenasanchez@gmail.es |
|
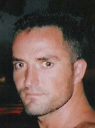 |
Eduardo Saez-Saez de Villarreal |
Employment: Professor at the Faculty of Sport – University of Pablo de Olavide, Spain. |
Degree: PhD |
Research interests: Strength and conditioning, neuromuscular fatigue and Biomechanics of human locomotor system. |
E-mail: esaesae@upo.es |
|
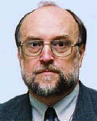 |
Mati Pääsuke |
Employment: Professor Dean of the Faculty of Exercise and Sport Sciences. University of Tartu. Estonia. |
Degree: PhD |
Research interests: Strength and conditioning, neuromuscular fatigue and Biomechanics of human locomotor system. |
E-mail: mati.paasuke@ut.ee |
|
|
|
REFERENCES |
 Aragon-Vargas L.F. (2000) Evaluation of four vertical jump tests: Metodology, reliability, validity, and accuracy. Measurement in Physical Education and Exercise Science 4, 215-228.
|
 Atkinson G., Nevill A.M. (1998) Statistical methods for assessing measurement error (reliability) in variables relevant to sports medicine. Sports Medicine 26, 217-238.
|
 Baker D. (1996) Improving vertical jump performance through general, special, and specific strength training: A brief review. Journal of Strength and Conditioning Research 10, 131-136.
|
 Bobbert M.F., Van Soest A.J. (2001) Why do people jump the way they do. Exercise and Sport Sciences Reviews 229, 95-102.
|
 Bosco C., Luhtanen P., Komi P.V. (1983) A simple method for measurement of mechanical power in jumping. European Journal of Applied Physiology an Occupational Physiology 50, 273-282.
|
 Casartelli N., Muller R., Maffiuletii A.M. (2010) Validity and reliability of the myotest accelerometric system for the assessment of vertical jump height. Journal of Strength and Conditioning Research 24, 3186-3193.
|
 Coventry E., O’Connor K.M., Hart B.A., Earl J.E., Ebersole K.T. (2006) The effect of lower extremity fatigue on shock attenuation during single-leg landing. Clinical Biomechanics 221, 1090-1097.
|
 Cronin J.B., Hing R.D., McNair P.J. (2004) Reliability and validity of a linear position transducer for measuring jump performance. Journal of Strength and Conditioning Research 118, 590-593.
|
 Dowling J.J., Vamos L.J. (2003) Identification of kinetic and temporal factors related to vertical jump performance. Journal of Applied Biomechanics 9, 95-110.
|
 Garcia-Lopez J., Peleteiro J., Rodriguez Marroyo J.A., Morante J.C., Herrero J.A., Villa J.G. (2005) The validation of a new method that measures contact and flight times during vertical jump. International Journal of Sports Medicine 26, 294-302.
|
 Hasan S.S., Robin D.W., Szurkus D.W., Ashmead D.H., Peterson S.W., Shiavi R.G. (1996) Simultaneous measurement of body center of pressure and center of gravity during upright stance. Part I: Methods. Gait Posture 4, 1-10.
|
 Hatze H. (1998) Validity and reliability of methods for testing vertical jumping performance. Journal of Applied Biomechanics 114, 127-140.
|
 Hopper D.M., Goh S.C., Wentworth L.A., Chan D.Y.K., Chau J.H.W., Wootton G.J., Strauss G.R., Boyle J.J.W. (2002) Tes-retest reliability of knee rating scales and functional hop tests one year following anterior cruciate ligament reconstruction. Physical Therapy in Sport 3, 10-18.
|
 Innocenti B., Facchielli D., Torti S., Verza A.S. (2006) Analysis of biomechanical quantities during a squat jump: Evaluation of a performance index. Journal of Strength and Conditioning Research 20, 709-715.
|
 Isaacs L.D. (1998) Comparison of the vertec and just jump systems for measuring height of vertical jump by young children. Perceptual and Motor Skills 86, 659-663.
|
 Klavora P. (2000) Vertical-jump test: A critical review. Strength and Conditioning Journal 22, 70-74.
|
 Leard J.S., Cirillo M.A., Kastnelson E., Kimiatek D.A., Miller T.W., Trebincevic K., Garbalosa J.C. (2007) Validity of two alternative systems for measuring vertical jump height. Journal of Strength and Conditioning Research 21, 967-972.
|
 Linthorne N.P. (2001) Analysis of standing vertical jumps using a force platform. American Journal of Physics 69, 1198-1204.
|
 Mayagoitia R.E., Nene A.V., Veltink P.H. (2002) Accelerometer and rate gyroscope measurement of kinematics: an inexpensive alternative to optical motion analysis systems. Journal of Biomechanics 335, 537-542.
|
 Musayev E. (2006) Optoelectronic vertical jump height measuring method and device. Measurement 339, 312-319.
|
 Quagliarella L., Sasanelli N., Belgiovine G., Moretti L., Moretti B. (2010) Evaluation of standing vertical jump by ankles acceleration measurement. Journal of Strength and Conditioning Research 24, 1229-1236.
|
 Rahmani A., Dalleau G., Viale F., Hautier C.A., Lacour J.R. (2000) Validity and reliability of a kinematic device for measuring the force developed during squatting. Journal of Applied Biomechanics 116, 26-35.
|
 Reiser R.F., Rocheford E.C., Armstrong C.J. (2006) Building a better understanding of basic mechanical principles through analysis of the vertical jump. Strength and Conditioning Journal 28, 70-80.
|
 Schmidtbleicher D., Komi, P (1992) The Encyclopedia of Sports Medicine. Training for power events. UK Blackwell. Oxford.
|
 Sleivert G., Taingahue M. (2004) The relationship between maximal jump-squat power and sprint acceleration in athletes. European Journal of Applied Physiology 91, 46-52.
|
 Thompson C.J., Bemben M.G. (1999) Reliability and comparability of the accelerometer as a measure of muscular power. Medicine and Science In Sports and Exercise 331, 897-902.
|
 Veltink P-H., Slycke P., Hemssems J., Buschman R., Bultstra G., Hermens H. (2003) Three dimensional inertial sensing of foot movements for automatic tuning of a two-channel implantable drop-foot stimulator. Medical Engineering and Physics 25, 21-28.
|
 Wilson G.J., Walshe A.D., Fisher M.R. (1997) The development of an isokinetic squat device: Reliability and relationship to functional performance. Journal 775, 455-461.
|
 Winter D.A. (1990) . Biomechanics and motor control of human movement , -.
|
 Ziv G., Lidor R. (2010) Vertical jump in female and male volleyball players: a review of observational and experimental studies.. Scandinavian Journal of Medicine and Science in Sports 20, 556-567.
|
|
|
|
|
|
|