|
|
|
ABSTRACT |
Despite the limitations set by FINA regulations, execution technique in breaststroke swimming is being improved thanks to more and more advanced analyses of the efficiency of the swimmer’s movements. The aim of this study was to detect the parameters of the time structure of the cycle correlated with the maximal swimming speed at the of 50 meters distance, in order to focus to specific technical aspects in the breaststroke training. In the group of 23 participants, between the age of 15.0 ± 1.17, the breaststroke cycle movement of the arms and legs was divided into two phases: propulsive or non-propulsive. In addition, indices characterizing the temporal coordination of movements of the upper limbs in relation to the lower limbs were distinguished: 1) Arm-Leg Lag - determines the interval between the phases of propulsion generated by upper and lower limbs; 2) Glide or Overlap - the inter-cyclic glide or overlap of the propulsive movement of the upper on lower limbs. Significant dependence was noted between the swim speed (V50surface breast) and the percentage of time of the arm propulsive in-sweep phase 0.64, p < 0.01. A significant correlation was observed between the V50surface breast with the percentage of partially surfaced hand phase of arm recovery 0.54, p < 0.01. Correlation between total leg propulsion and non-propulsion phases with V50surface breast was 0.49 and -0.49 respectively, both p < 0.01. The Glide or Overlap index was significantly related to the swimming speed V50surface breast 0.48, p < 0.05. This type of analysis suggests how to refine the swimming technique, with the goal to improve the current speed capabilities; furthermore the results also indicate the direction of its development in the future swimmers of the group studied. |
Key words:
Swimming technique, video analysis, propulsion efficiency
|
Key
Points
- This study investigated the influence of the inter- and intra-cyclic time structure of the movements in sprint breaststroke swimming.
- The distinction of the operations phases of the upper limbs in the propulsive movement shows significant correlation 0.64, p <0.01 between the swimming speed V50 and the execution time of the in-sweep phase in the movement cycle. Significant relationship was noted between minimizing the first non-propulsive phase of arm recovery with higher contribution of the next, partially immersed sliding phase of arm recovery.
- The specification of the inter-cyclic coordination index of the upper and lower limbs during the movement cycle shows influence of the overlap of the propulsive movement of the upper limbs on the propulsive movement of the lower limbs on V50 with correlation 0.48, p <0.05 for young swimmers.
|
In recent two-three decades analysis of breaststroke swimming has been conducted by using video recordings, where in the movements of the upper and lower limbs were generally distinguished into three phases: propulsive, resistant and gliding. The movement technique was also analyzed by examining the use of wavy body movements (Colman et al., 1998; Persyn et al., 1992; Vilas-Boas, 1996). In these and other articles (Barbosa et al., 2008; Chollet et al., 2004; Leblanc et al., 2005; 2009; Seifert and Chollet, 2005; Seifert et al., 2010; 2011; Takagi et al., 2004) various quantitative indicators of the complex coordination of movements of the upper limbs in relation to the lower limbs were provided along with a study of the distribution of these movements in the propulsive and non-propulsive phase, for the purpose of analyzing the best efficiency while swimming. An overview of the work shows the possibility for the application of coordination mode measurement occurring inside a movement cycle with details of propulsive and non-propulsive phases in movement of pairs of limbs. We also believe that the assumptions developed for the establishment of (IdC) coordination index (Chollet et al., 2004) will be helpful in detection of temporal structure of breastroke cycles in particular a description of the movements in the case of occuring the inter-cyclic gliding phase or lack of it between cycles or coordination with cycle overlapping. Researches were designed to analyze the association between several variables of the time structure of the breaststroke cycle as well as to check influence of those technical indices on the breaststroke swimming. The distinction of the operations of the upper and lower limbs during the movement cycles in the propulsive and non-propulsive phases will allow for the specification of the intra-cyclic and inter-cyclic coordination of the movements in sprint breaststroke swimming. Further, the main aim was to detect the parameters of the time structure of the cycle correlated with the maximal swimming velocity in order to focus to specific technical aspects in the breaststroke training. Collecting such data, regarding the shaping of the swimming technique, will allow for an examination the hypothesis of a relationship between the specific cycle indicators of propulsive and non-propulsive movement and their influence on sprint swimming speed for a distance of 50 meters achievable by young swimmers.
Participants Volunteers were recruited from two sports schools. An informed consent form (approved by the Bioethics Commission in Cracow) was signed by either the participant or his parents. The 23 male swimmers, from either the regional or national level, trained twice a day six times a week. They were 15.0 ± 1.17 years old: between the age of 14 to 17 years. Their average height was 1.79 ± 0.07 m, and body mass 68.5 ± 9.10 kg. The swimmers specialized in the breaststroke as well as in individual medley swimming. Their average result in the 50m all-out breaststroke swimming speed test carried out in a 25 meter-long was 1.43 ± 0.08 m·s-1.
Underwater recordings The underwater swimmers’ body movements were recorded using a Canon Legria HV40 (Japan) camcorder and an underwater camera, Sony Color Submergible Camera IP:68 (Japan). The camcorder was in recording mode, receiving a picture signal from underwater camera. Video images were recorded at a sampling rate of 50Hz. The camcorder and underwater camera were installed on a portable trolley, which moved along the swimming pool border and parallel to the subject, providing sagittal images of the swimmer. A person trained in maintenance of the above mentioned device moved the trolley in both directions alongside the swimming pool so as not to cross, with the lens of the underwater camera, the perpendicular lines between the swimmer’s fingertips of the straightened arms in the front and toes of the stretched legs in the back. The underwater camera was mounted to the lower arm of trolley and submerged in water about 1 meter below the surface and approximately 5 meters from the swimmer’s lane.
Swimming testThe all-out swimming 50m breaststroke speed test was carried out in a 25 meter-long swimming pool with similar conditions as in a competition (e. g. start was from the block, swimming without any drafting effect). The test was preceded by a warm-up, similar to the one performed before a competition.
Video analysis of breaststroke swimmingThis enabled the determination of the moments in which characteristic events in the swimming cycle occurred, namely specific arm and leg movements. The cyclic movement of the arms and legs was divided into phases according to its role in accelerating the swimmer’s body (propulsion or non-propulsion phase). Identification of the various phases of the movement was based on the modified method proposed by Maglisho, 2003. Three arm propulsion phases were determined: first phase - AP1 starts from the beginning of the arm movement toward the outside with the hands twisted out in pronation, and lasts until the elbows are bent outwards and move back; second phase - AP2 begins with the end of the AP1 phase with the trough arm pull, a backward movement leading to the deepest immersion of the fingertips, which are moving inward; third phase - AP3 starts at the end of the AP2 phase and lasts till the beginning of forward hand movement. The arm recovery (Total AR): We can assume that the swimmers will recover their hands either emerged or fully submerged. Therefore, we at least want to highlight the partially emergent hand phase called ARair and the fully submerged arm phase ARwater. Arm recovery phases ARair and ARwater were determined as follows: first phase - ARwater 1 from the end of the AP3 phase till hand emersion (emergent hands become invisible); second phase - ARair from the end of the ARwater 1 phase till hand submersion; third phase - ARwater 2 from the end of the ARair phase till the beginning of AP1. If the ARair phase did not occur, the swimmer obtained 0% contribution to this phase in the cycle according to the observation. If there was hand emergence then ARwater = ARwater 1 + ARwater 2. Conversely, if there was no emergence, then ARwater = Total AR. Two leg propulsion phases were determined: first phase - LP1 after bending the legs at the hip and knees, the phase begins with the ankles moving backwards and ends when the knee straightens; second phase - LP2 from the end of the LP1 phase till the closure of feet approximation. Two leg recovery phases were identified: first phase - LR1 starts at the end of the LP2 phase and ends when the swimmer bends the leg in the hip and knee while pulling feet towards the hips; second phase - LR2 from the end of LR1 till the ankles begin to move backward. Data collected over the course of time was recorded in a spreadsheet and converted into graphs for each swimmer as presented in the results section in Figure 1. Based on the collected data on the percentage of the execution time of the respective phases, two indicators, which distinguish the temporal propulsive movement coordination of the upper and lower limbs were reported: 1) Arm-Leg Lag - the intra-cyclic gap between the propulsive phases produced by the upper and lower limbs. The time between the end of arm propulsion i.e. end of AP3 phase till the beginning of leg propulsion i.e. LP1 phase; 2) Glide or Overlap - the inter-cyclic glide or overlap of the propulsive movement of the upper limbs on the propulsive movement of the lower limbs of the previous cycle, as a certain percentage of the movement cycle of the upper and lower limbs. If the propulsive phases overlapped then the inter-cyclic index was positive (e.g. after arm propulsion still executing leg propulsion LP2 or LP3 with simultaneously beginning next arm stroking in the new cycle - AP1 or AP2); when glide phase occurred then the index took negative values. The analysis of video recordings was conducted using the above-mentioned identification of the characteristic cyclic elements of arm and leg movements in breaststroke swimming. The analysis during the all-out swimming test was conducted in 10 m sectors, each 10m sector on each 25m pool (short course) of the distance of 50 meters - respectively in the sections between the 10th to 20th meter and between the 35th to 45th meters. The duration of the race ∆ti and the times of the separate sectors were measured by an expert evaluator using stopwatch with the accuracy of 0.01 s. The following parameters were used to assess the swimming technique during each analyzed 10m-long swim (i=1,2): 1) Swimming speed: Vi = 10m / ∆ti, [m·s-1], 2) Stroke rate (SRi): calculated as the reciprocal of the arithmetical average of the duration of three analyzed swimming cycles: SRi = 1/Ti, [cycle·min-1], 3) Stroke length (SLi), calculated as the average speed to SRi ratio: SLi = Vi/SRi [m]. Results of the swimming technique parameters SR and SL as well as the kinematic indices described above are presented as an average calculated based on six full movement cycles of the legs, from which 3 were identified between the 10th to 20th meter sector, and the next 3 between the 35th to 45th meter section of the total 50 meter distance.
Statistical analysisThe normality, homoscedasticity and independency of data assumptions were checked respectively with Kolmogorov-Smirnov, the Levene and Durbin-Watson tests. Descriptive statistics of means and standard deviations were calculated for all variables. The partial correlations, controlled for age were conducted between the swimming speed (V50surface breast) and the respective arm and leg propulsion and recovery phases as well as indexes of cycle coordination (Arm-Leg Lag, Glide or Overlap) indexes or swimming stroke kinematics SR and SL. Once again the partial correlations, controlled for age, were computed between coordination indexes Arm-Leg Lag, Glide or Overlap, basic breaststroke stroke kinematics SR and SL and total propulsive and non-propulsive phases of arm and leg movements. Pearson correlation was computed between Arm-Leg Lag and Glide or Overlap indexes. All tests were conducted with TATISTICA 6.1 software (StatSoft, Inc). The signifycance level was set at p < 0.05.
Mean breaststroke surface swimming speed (excluding start and turn zones) was 1.23 ± 0.08 m·s-1. Figure 1 shows the average movement cycle containing all of the indicated propulsive and non-propulsive phases of the upper and lower limbs in relation to each other during the movement cycle of a select breaststroke swimmer tested at a distance of 50 meters. The beginning of the movement cycle was arbitrarily determined in accordance with the FINA rules, which state that at the beginning and during the race the stroke cycle is one arm stroke and one leg kick, in that specific order. The index Glide or Overlap was significantly positively associated with the V50surface breast (Table 1). The stroke rate variable reached an average level of correlation with V50surface breast, but it was statistically negligible as it happens with LP2 and LR2 (Table 5) or total AP and total AR (Table 4). The Glide or Overlap index defined, in one integer, both the size of the observed gliding and presence of the propulsive movement overlap. Gliding was observed in 16 swimmers and in 5 the overlap of the AP1 phase on the LP1 phase, which originated from previous movement cycle. Similarly in 1 of the swimmers the overlap of AP2 phase onto LP2 phase was found, and in one case, it was a continuous alternating propulsion movement (no gliding). The Glide or Overlap index was highly and positively associated to the SR indicator and negatively associated on the verge of significance with SL (Table 2). Moreover, the Glide or Overlap index was linearly positively (0.64, p = 0.001) related to the Arm-Leg Lag index. Following the interplay of Arm-Leg Lag, Glide or Overlap with the total percentage of the propulsion and non-propulsion phases of upper and lower limbs was specified with age control. Positive interplay between the two coordination indices and the percentage of Total LP phase and the negative interplay to the Total LR were noted (Table 3). Similar interplay between the Glide or Overlap index and Total AP or Total AR was shown (Table 3.). The arm propulsion and recovery phases of breaststroke swimming and their partial correlations with swimming speed - V50surface breast with age control are shown in Table 4. Per cent duration of the AP3 phase of Arm-Propulsion was significantly positively associated with V50surface breast. In the total hand recovery separated ARair phase was significantly positively associated with V50surface breast (Table 4), while the percentage of recovery time in immersion ARwater had a negative impact on V50surface breast. A percentage of Leg-Propulsion phases correlated significantly with V50surface breast. The interrelationship of V50surface breast and the phase LR1 of Leg-Recovery, which is generally connected to body gliding was high and negative (Table 5) In the operations of lower limbs, the lack of the LP2 phase in some swimmers gave the result of 0% in this phase.
The main aim of this research was to detect the parameters of the time structure of the cycle correlated with the maximal swimming velocity in order to focus to specific technical aspects in the breaststroke training, including assessment of the influence of a given coordination on swimming speed. Contrary to the well known in literature TTG index (Seifert and Chollet, 2005) which is the sum of the different time gaps between arm and leg actions, the coordination for each swimmer was described using two indices: Arm-Leg Lag - measuring the intra-cyclic limb propulsion gaps: this means first using upper extremities, gap, then using lower extremities; and: Glide or Overlap - measuring both propulsion gap, which is called gliding occurring after leg movements, normally longer for long distances, or, if it does not occur, is shows overlapping of the arm movement from the new cycle and leg movement from the previous cycle. One of the main finding of this study was that the reduction of the glide and even overlap of the propulsive movements of the upper and lower limbs described by Glide or Overlap index significantly influenced the swim speed - V50surface breast. Although the level of Glide or Overlap index explains the occurence of the continuity or discontinuity in propulsion generation between cycles, on the other hand it may no longer be so obvious that its level has an effect on of the increase in the propulsion percentage in the movement cycle; however, the Glide Overlap index showed a strong dependence with in Total AP and Total Total LP. These strong associations may be a multicolinerity phenomenon, because coordination indexes are computed in some part with phase durations, but we wanted to show them in shaping of the cycle. In the researches of Leblanc et al. (2005; 2007), the effective propulsion times of the elite groups were longer and their effective glide times shorter than non-elite swimmers. Indeed, long before D’Acquisto and Costill (1998) claimed that by utilizing overlap timing and better body position, the period of deceleration can be reduced and higher minimum linear body velocity before the propulsive phase generated from the arm pull established, and sprinting ability enhanced. Besides this fact, showing the results in Table 1, we consider important Maglisho’s (2003, p. 244) statement that: Swimmers are taught to wait until the legs come together before they begin sweeping the arms out." According to him, during the gliding phase of a chosen swimmer lasting about 0.40 s, a swimmers’ velocity declines from 1.60 m·s-1 to about 1.22 m·s-1. This author also points out that the best way to reduce the time of deceleration is to use overlap timing between the end of the propulsive phase of the kick and the beginning of the propulsive phase of the armstroke (Maglisho, 2003). In our research this learned method of movement coordination was noticeable, with the glide occurring before the next outsweep of the arm was visible; on the other hand we also registered the overlapping of the arm outsweep on the leg propulsive movements, but only in 6 swimmers. The simultaneously high association of the Glide or Overlap index with SR (Table 2) indicates that the quick start of the production of propulsive force by using the upper limbs to pull consecutively was a method to produce increased propulsion, and the average SR in the studied group, which was less than 47 cycles per minute shows a great potential to increase the amount of movement cycles. An example of successfully executed movements with SR 57 cycles per minute by one of the best swimmers in the examined group is shown in Figure 2. Expansion on the results that we were able to ob-serve is the data from the European Junior Swimming Championships in Beograd in 2011 wherein the men breaststroke 50m final ended with the average SR equal to 64.5 cycle·min-1 (Haljand, 2012), which may indicate that those capable swimmers achieved high speed, using SR close to the maximal level. This is why top level, professional swimmers, with the best speed abilities, swimming breaststroke for 50 meters, for example finalists during World Swimming Championships in Fukuoka in 2001 demonstrated lower overlapping propulsion movements of the arms and legs alongside with higher SR exceeding 60 cycle·min-1. Most importantly, they showcased lower overlapping possessing longer SL up to 1.6 m. The differences between the finalists and the eliminated breaststrokers were taken note of by Takagi et al., 2004. Very high partial correlations obtained in Table 3. between Glide or Overlap with Total LP, LR and Total AP, AR may be explained by the fact that they have similar effect on V50surface breast (Total AP, AR nosignificant), and they result from the same movement cycles. Our aim was to show the dependencies determining swimming speed, which consists of movements in each cycle. Barbosa et al., 2010b in their study on deterministic models in competitive swimming show that high-level swimmers are characterized by lower total time gap (TTG) -inter limb coordination. That lowering in the percentage of non-propulsive phases in the cycle movement (in simple way) changes to the direction of increasing the propulsion phases (Leblanc et al., 2005; 2007; 2009). In other research Barbosa et al., 2010a have noted strong dependencies, a little bit weaker than in our research (Table 3), between the indices characterizing swimming style such as stroke length, stroke index and propulsive efficiency, despite the fact the indices were calculated in different ways. In this study, creation of the Arm-Leg Lag index was supported by the recommendations of Haljand, 2012, he has advised breastrokers that "the timing of stretching of arms and final flexing of legs must be done quickly, without the body dropping before new kick". In our study faster start of leg propulsion expressed by the Arm-Leg Lag index was also associated with the percentage of duration time of leg propulsion (Total LP) in each kick. Probably more time spent in Arm-Leg Lag by some swimmers allowed them to complete more ample recovery, as bringing their lower leg to the buttocks might give their feet a better starting position for the next outsweep (Leblanc et al., 2005). The better starting postion could help in clarifying the catch, limiting slipping in all propulsion phase from the leg. In this study it was noted that in sprint swimming there is significant correlation between the swimming speed V50surface breast and the execution time of the intra-cyclic propulsion AP3 phase in the movement cycle (Table 3). This aspect of the propulsion, where mainly the hands and forearms move toward each other in a propeller-like movement, is called the in-sweep phase. The propeller-like pulling pattern theory, presumes that efficient pulling actions use complex movements so that at every point of the pull the working segments of the arms interact with standing, immobile water (Vorontsov and Rumyantsev, 2000). It is explained that these conditions are fulfilled when the pulling actions are not exactly and linearly performed backwards, but rather when a complex curvilinear trajectory is employed, across the transverse (z-y) and the vertical (x-y) planes. Vorontsov and Rumyantsev, 2000 state that in using transverse and vertical sculling movements, swimmers achieve a high magnitude of lift (directed forward) without significant displacement of water mass backwards, prolonging the duration of action of the propulsive force. Tourny et al. (1992) in their study stated for example that the main propulsive effect in breaststroke generally occurred during the insweep of the arms or during the second half of the outsweep. Our knowledge about the shaping of the movement patterns of the upper limbs may be consistent with the above mentioned citations, because as we know, learning effective sculling patterns requires talent in sensing and executing the most effective movement and training aimed at producing lift force and sculling in reciprocating motion. Most of this lift force is produced by internal rotators and flexors of the arm musculature. During this phase of inward sculling, hands move with an efficient angle of attack, approximately 40° was observed in competitive swimmers (Schleihauf, 1979), and in a group of mixed level swimmers (Martens and Daly, 2012). Positive and strong interplay between the ARair phase and V50surface breast indicates that the ability to eliminate unnecessary resistance during transport, by sliding the upper limbs, partially immersed, and reducing deceleration, is a factor that enhances the average swim speed. Such sliding while simultaneously minimizing water resistance is faster than total immersion. In addition, a horizontal longitudinal movement of the hand can contribute to more linear movement of the body with reduced up-and-down movement and may induce earlier execution of a successive propulsive movement of the upper limbs and shorten their and body gliding with appropriately early hand pronation and catch. Some speculations can be addressed supported in previous findings for kickboard and breaststroke kick at the distance of 50 m (Strzala et al., 2012). We have noted significant dependence 0.56, p < 0.01., in the partial correlation measurements with age control, between V50surface breast and the average 50 m swimming speed using a kickboard and breaststroke kick only (from above mentioned study). This result confirms the great influence of the lower limbs on overall propulsion in the breaststroke. As it was shown in Vilas-Boas, 1994 observation where maximum breaststroke propulsive impulses were associated with the leg kick phase, this also demonstrates the group differentiation in this matter. In this study, the overall contribution of time in producing a propulsive force by the lower limbs in the movement cycle significantly influenced the results of V50surface breast (Table 5). From our observations (Strzala et al., 2012) as well as from other researchers’ observations (Jagomägi and Jürimäe, 2005; Kippenhan, 2002), it follows that this complex multidimensional breaststroke kick, also called the whip kick (Kippenhan et al., 2001; 2002), dependent on the knee’s external rotation, may be executed more extensively. Increase in the range of the movement as well as prolongation of the duration of leg propulsive force across the transverse (z-y) and vertical (x- y) planes increased the instantaneous forward displacement of the torso (Vorontsov and Rumyantsev, 2000). This was observed in the research of Chollet et al., 2004 for example, and measured by using the swim speedometer in this phase of the cycle. The contribution of the lower extremities’ non-propulsive phases was correlated to V50surface breast to the same extent but negatively. This was mainly due to gliding, even if in a well-streamlined position; however, the longer the glide, the more the minimal contribution of the propulsive phases in the movement cycle. Along with these observations, we have not considered the leg and torso movement track during the LR1 gliding phase more deeply, but some researchers point out that the amplitude of undulating hip and feet movements may contribute to enhanced resistance production, which also results in lower economics (Vilas-Boas, 1996). So, during the in-sweep phase of the foot movement, hips are pushed too high up, and then during the undulating glide, the feet follow the hips, particularly using the “Hip Wave Undulation With Feet Breaking Surface" swimming technique (Kilani et al., 2007). This resistance increases as the body is dragged in the water.
In this study the hypothesis of the influence of inter- and intra-cyclic movement time structure of breaststroke technique on the 50-m race performance was accepted. The trained swimmers subjected to the observations could do effective propulsive movements resulting in the achieved higher swimming speed by adjusting movement coordination of the proper extremities with shorter or lack of inter-cyclic glide or overlapping propulsive arm-leg action resulting higher Glide or Overlap index. Do not forget that increasing the continuity of propulsion production using his method should be accompanied by increase in percentage of propulsive phases and retaining of proper distance travelled in each cycle. The structure of intra-cyclic upper extremities movement, especially ample AP3 phase (insweep phase) strongly associated with V50surface breast, shows that better swimmers have been shaping more efficient technique. The slower, however, avoided common mistakes such as push down and later back against the water with the dropped elbow position which would also make the AP3 phase longer and also lowering the interplay with V50surface breast. In this situation shortened non-propulsive phase, underwater arm-recovery phase, may be obtained.
ACKNOWLEDGEMENTS |
The authors would like to thank the swimmers and their coaches for their commitment during this study. |
|
AUTHOR BIOGRAPHY |
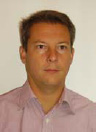 |
Marek Strzala |
Employment: Department of Theory and Methodology of Water Sports, University School of Physical Education, Cracow, Poland |
Degree: PhD |
Research interests: Exercise physiology, swimming biomechanics, swimming performance analysis |
E-mail: marek.strzala@awf.krakow.pl |
|
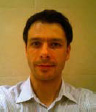 |
Piotr Krezalek |
Employment: Movement Analysis Laboratory in the Department of Physiotherapy Faculty of Motor Rehabilitation, University School of Physical Education, Cracow, Poland |
Degree: MSc |
Research interests: Biomechanics of sport and rehabilitation, human movement technique analysis and performance improvement in pathological gait and various sports, especially ski jumping and swimming |
E-mail: piotr.krezalek@awf.krakow.pl |
|
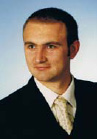 |
Grzegorz Glab |
Employment: Department of Physiotherapy, University School of Physical Education, Cracow, Poland |
Degree: PhD |
Research interests: Exercise physiology, sports medicine, physical therapy |
E-mail: grzegorz.glab@awf.krakow.pl |
|
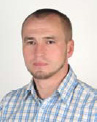 |
Marcin Kaca |
Employment: Department of Theory and Methodology of Water Sports, University School of Physical Education, Cracow, Poland |
Degree: PhD |
Research interests: Lifesaving, water safety prevention, coaching sciences |
E-mail: marcin.kaca@awf.krakow.pl |
|
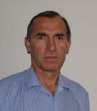 |
Andrzej Ostrowski |
Employment: Department of Theory and Methodology of Water Sports, University School of Physical Education, Cracow, Poland |
Degree: PhD |
Research interests: Coaching sciences, swimming performance analysis, freediving |
E-mail: andrzej.ostrowski@awf.krakow.pl |
|
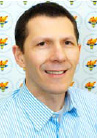 |
Arkadiusz Stanula |
Employment: Department of Sports Training,The Jerzy Kukuczka Academy of Physical Education, Katowice, Poland |
Degree: PhD |
Research interests: Coaching sciences, swimming performance analysis, freediving |
E-mail: a.stanula@awf.katowice.pl |
|
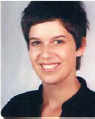 |
Anna K. Tyka |
Employment: Department of Recreation and Biological Regeneration, University School of Physical Education, Cracow, Poland |
Degree: PhD |
Research interests: Time of the day effects on different exercise abilities, circadian rhythms, sports physiology |
E-mail: anna.tyka@awf.krakow.pl |
|
|
|
REFERENCES |
 Barbosa T.M., Bragada J.A., Reis V.M., Marinho D.A., Carvalho C., Silva A.J. ((2010b)) Energetics and biomechanics as determining factors of swimming performance: updating the state of art. . Journal of Science and Medicine in Sport 13, 262-269.
|
 Barbosa T.M., Costa M., Marinho D.A., Coelho J., Moreira M., Silva A.J. ((2010a)) Modeling the links between young swimmers’ performance: energetic and biomechanic profiles. . Pediatric Exercise Science 22, 379-391.
|
 Barbosa T.M., Fernandes R.J., Morouco P., Vilas-Boas J.P. ((2008)) Predicting the intra-cyclic variation of the velocity of the centre of mass from segmental velocities in butterfly stroke: A pilot study.. Journal of Sports Science and Medicine 7, 201-209.
|
 Chollet D., Seifert L., Leblanc H., Boulesteix L., Carter M. ((2004)) Evaluation of the arm-leg coordination in flat breaststroke.. International Journal of Sports Medicine 25, 486-495.
|
 Colman V., Persyn U., Daly D., Stijnen V. ((1998)) A comparison of the intra-cyclic velocity variation in breaststroke swimmers with flat and undulating styles. . Journal of Sports Sciences 16, 653-665.
|
 D’Acquisto L.J., Costill D.L. ((1998)) Relationship between intra-cyclic linear body velocity fluctuations, power, and sprint breaststroke performance. . Journal of Swimming Research 13, 8-14.
|
 Haljand R. ((2012)) . http://www.swim.ee/competition/2011_beograd/mbre50f.html
|
 Jagom gi G., J rim e T. ((2005)) The influence of anthropometrical and flexibility parameters on the results of breaststroke swimming. . Anthropologischer Anzeiger 2, 213-219.
|
 Kilani H., Al-Kilani M., Duka B., Menzel H.-J., Chagas M.H. ((2007)) Kinematical analyses of two styles in breast strokes swimming.. ISBS - Conference Proceedings Archive, XXV International Symposium on Biomechanics in Sports, ISSN 1999-4168. International Society of Biomechanics in Sports, Ouro Preto - Brazil , 196-199.
|
 Kippenhan C.B., Blackwell J.R., Sanders R.H. ((2001)) Proceedings of swim sessions: XIX International Symposium on Biomechanics in Sports. Influence of lower extremity joint motions of the effectiveness of the kick in breaststroke swimming.. San Francisco, CA. University of San Francisco.
|
 Kippenhan C.B., Gianikellis K.E. ((2002)) Lower-extremity joint angles used during the breaststroke whip kick and the influence of flexibility on the effectiveness of the kick.. ISBS - Conference Proceedings Archive, XX International Symposium on Biomechanics in Sports, ISSN 1999-4168. International Society of Biomechanics in Sports, C ceres - Spain , 31-34.
|
 Leblanc H., Seifert L., Baudry L., Chollet D. ((2005)) Arm-leg coordination in flat breaststroke: a comparative study between elite and non-elite swimmers. . International Journal of Sports Medicine 26, 787-797.
|
 Leblanc H., Seifert L., Chollet D. ((2009)) Arm-leg coordination in recreational and competitive breaststroke swimmers. . Journal of Science and Medicine in Sport 12, 352-356.
|
 Leblanc H., Seifert L., Tourny-Chollet C., Chollet D. ((2007)) Intra-cyclic distance per stroke phase, velocity fluctuations and acceleration time ratio of a breaststroker’s hip: a comparison between elite and non-elite swimmers at different race paces.. International Journal of Sports Medicine 28, 140-147.
|
 Maglisho E.W. ((2003)) . Swimming fastest.. Human Kinetics. Leeds.
|
 Martens. J., Daly D. ((2012)) Qualitative evaluation of water displacement in simulated analytical breaststroke movements. . Journal of Human Kinetics 32, 53-63.
|
 Persyn U.J., Colman V., Van Tilborgh L., MacLaren D., Reilly T., Less A. ((1992)) Swimming Science VI. Movement analysis of the flat and undulating breaststroke pattern.. London, England.
|
 Schleihauf R.E., Terauds J., Bedingfield E.W. ((1979)) Swimming III. A hydrodynamics analysis of swimming propulsion.. Baltimore, MD. University Park Press.
|
 Seifert L., Chollet D. ((2005)) A new index of flat breaststroke propulsion: comparison between elite men and elite women. . Journal of Sports Sciences 23, 309-320.
|
 Seifert L., Leblanc H., Chollet D., Delignieres D. ((2010)) Inter-limb coordination in swimming: effect of speed and skill level. . Human Movement Science 29, 103-113.
|
 Seifert L., Leblanc H., Herault R., Komar J., Button C., Chollet D. ((2011)) Inter-individual variability in the upper-lower limb breaststroke coordination. . Human Movement Science 30, 550-565.
|
 Strzala M., Kr?zalek P., Kaca M., Glab G., Ostrowski A., Stanula A., Tyka A. ((2012)) Swimming speed of the breaststroke kick.. Journal of Human Kinetics 35, 133-139.
|
 Takagi H., Sugimoto S., Nishijima N., Wilson B. ((2004)) Differences in stroke phases, arm-leg coordination and velocity fluctuation due to event, gender and performance level in breaststroke. . Sports Biomechanics 3, 15-27.
|
 Tourny C., Chollet D., Micallef J.P., Macabies J., MacLaren D., Reilly T., Less A. ((1992)) Swimming Science VI. Comparative analysis of studies of speed variations within a breaststroke cycle.. London. E & FN SPON.
|
 Vilas-Boas J.P., Barab s A., F bi n Gy ((1994)) Maximum propulsive force and maximum propulsive impulse in breaststroke swimming technique.. ISBS - Conference Proceedings Archive, XII International Symposium on Biomechanics in Sports, ISSN 1999-4168. International Society of Biomechanics in Sports, Budapest-Si fok, Hungary , 303-310.
|
 Vilas-Boas J.P., Troup J.P., Hollander A.P., Strasse D., Trappe S.W., Cappaert J.M., Trappe T.A. ((1996)) Swimming Science VII. Speed fluctuations and energy cost of different breaststroke techniques.. London, England. E & FN SPON.
|
 Vorontsov A.R., Rumyantsev V.A., Zatsiorsky V. ((2000)) The Encyclopaedia of Sports Medicine. Resistive forces in swimming.. London. Blackwell Science Ltd.
|
|
|
|
|
|
|