|
|
|
ABSTRACT |
Heart rate (HR) was tested as a reliable index for recovery management during interval training (IT), considering its relationship with the several factors involved in respiratory, metabolic and cardiovascular homeostasis. Thirteen runners underwent two different IT sessions: at 80% and 120% of the second ventilatory threshold (VT2). Throughout both sessions HR, oxygen uptake (VO2), carbon dioxide production (VCO2) and pulmonary ventilation (VE), were measured by means of a portable gas analyzer. Carbon dioxide production excess (CO2excess), respiratory exchange ratio (RER), oxygen pulse (OP) and oxygen debt (O2debt) were also estimated. A significant increase in HR values (144 versus 150 beats·min1 between the first recovery and the last, p < 0.001) was observed at 80% of the VT2 speed. At the over-threshold intensity, HR rose from 159 to 168 beats·min1 from the first recovery to the last (p < 0.001). OP showed a declining trend from the first to the last recovery at 80% at the VT2 speed (18.3 versus 16.4 mL·beats1, p < 0.05) and between the first and the last recovery in tests performed at 120% of the VT2 speed (17.8 versus 16.3 mL·beats1, p < 0.05). No change occurred in CO2excess, VO2, RER, VE and O2debt. On the basis of our research, the use of fixed HR as a reliable index of the established recovery is inaccurate and unfit for training. The phenomenon of cardiac drift to set the restart timing after the repetitions, i.e. by progressively increasing HR values, should be taken into account by coaches. |
Key words:
Carbon dioxide excess, oxygen pulse, oxygen debt
|
Key
Points
- During an IT session, if recovery time after repetitions is fixed, HR supplies a different indication compared to all the respiratory parameters: HR indicates an incomplete recovery while the other parameters do not.
- The use of fixed HR values as a reliable index of the established recovery during IT is inaccurate and it may be the cause of under-training.
- To set the restart timing after repetitions the phenomenon of cardiac drift should be taken into account by coaches.
- HR drift during recoveries did not appear linked to the CO2excess.
|
Although athletes spend a greater amount of time resting than performing, research has dedicated little attention to the practical management of functional recovery after training (Bishop et al., 2008). Complete restoration of the integrity of the organs stressed by workloads is essential to attain optimal performance and this can be achieved only through an appropriate balance between loads administered, physical and psychological stress deriving from the competition and functional recovery. Thus there is a wide variety of recovery techniques as part of training programs targeting this goal (Barnett, 2006). For such reasons, some scientific updates on this topic have recently highlighted the need to expand studies on various aspects of functional recovery in athletes (Bishop et al., 2008; Barnett, 2006; Achten and Jeukendrup, 2003). Functional recovery can be divided into three main categories: a) immediate relief after a workout, b) short recovery between repetitions during a session of interval training, c) recovery practices between workouts (stretching, massage, sauna etc). The focus of this research was to address point b) of the aforementioned classification in an attempt to add to current knowledge on metabolic adjustments that occur during the short recovery between repetitions in interval training (IT) in athletics. In most cases coaches manage the recovery between repetitions by selecting one of three empirical procedures: (i) a ratio between exercise and recovery times. If one has a 3min workout, according to the energetic pathway to elicit, coaches set a recovery (e.g. 1:1, meaning that recovery will take 3min as well); (ii) monitoring the RPE or any other subjective way to learn how tired the athlete is; (iii) using an HR value as a percentage of the theoretical maximum (HRmax, calculated as 220 beats minus age): the athlete is considered ready to restart an effort when an empirically established percentage value of HRmax is restored. An itemized focus on the prescription of IT with a fourth solution for determining duration and intensity of recovery time was recently pointed out by Tschakert and Hofmann (2013). In their brief review the authors suggested the use of a threshold model rather than percentages of VO2max or HRmax to set the exercise (Ppeak) and recovery (Prec) workloads and the use of an equation (equation 1) to calculate the mean load (Pmean):
Pmean = (Ppeak · tpeak + Prec · trec)/(tpeak + trec) |
Eq 1 |
where tpeak and trec are the peak workloads and recovery durations respectively.
By using this method the authors of the aforementioned review made a good attempt to modulate the IT in accordance with the training periods and cardiorespiratory strain. Significant in this regard are the recent results by Tschakert et al. (2015) which showed that the use of % HRmax to prescribe exercise intensity in long IT resulted in different values for Pmean, Ppeak and Prec across athletes with respect to their individual workload at the second threshold measured in an incremental exercise test (IET). Therefore, with the combined use of a threshold model and equation 1 it would be possible to provide more accurate HR management. In any case, several practitioners, even when aware that HR is not an accurate way to manage recovery during IT, probably use HR because it is an affordable and straightforward way. However, recovery time management of this kind is experiencing difficulties: the time of the recovery of fixed HR values can increase depending on several factors (intensity and number of repetitions, weather conditions, hydration status etc.). This is to say that we are dealing with a phenomenon known as cardiac drift (Coyle, 1998; Goodie et al., 2000; Gonzà les-Alonso et al., 1997). Furthermore, both recovery load and recovery duration affect muscle metabolic recovery which is crucial in maximizing work capacity during the subsequent intervals (Bucheit and Laursen, 2013). Our research aimed to analyze the use of HR as a reliable index for recovery management during IT, considering its relationship with the several factors that can influence respiratory, metabolic and cardiovascular homeostasis and their implications in the cardiac drift phenomenon. Taking into account that the IT method was introduced to extend training duration at intensities higher than those achievable with continuous training, the most precise indication possible on recovery time should be established during IT sessions.
SubjectsThirteen national-level middle-distance runners (MDR) were recruited. The athletes' anthropometric and functional characteristics are summarized in Table 1. Before testing, all participants were informed of the risks associated with the study and written informed consent was obtained in accordance with the Helsinki Declaration. To ascertain the athletes’ good health and to exclude any cardiovascular or respiratory disease, all participants underwent medical evaluation.
DesignThe subjects attended 2 separate testing sessions at our laboratory over a 3-week period. Initially, they completed an IET on a treadmill (RunRace, Technogym, Italy) to establish each individual’s Ventilatory Threshold 2 (VT2, see also below) or “anaerobic threshold” according to the three-phase model (Binder et al., 2008) and maximum oxygen uptake (VO2max). The following day each subject performed two IT sessions at 80% and 120% of the VT2 speed in a randomized order and spaced at 6 hours. Before all testing, subjects were required to abstain from any other unaccustomed strenuous exercise for at least 24 hours (aside from our protocol). Subjects were recruited in agreement with their coaches, who were training them to evaluate the level of training reached and to ameliorate the training program and establish their individual running times. Inclusion criteria were no less than 14 h training per week. Testing was performed at the same time of day (± 2 h, to minimize diurnal variation), and on the same treadmill.
Incremental Exercise Test (IET)The athletes initially undertook an IET until exhaustion. Each athlete began running at a speed of 10 km·h1 followed by a gradual 1 km·h1 increase every 2 minutes, until exhaustion. The total distance covered was between 5000 and 6000 m. The ventilatory equivalent method was used to determine VT2 i.e., the respiratory compensation point was identified as the point at which the VE/VCO2 ratio began to increase (Meyer et al., 2004). In this way, it was possible to determine the two speeds for performing the IT sessions, i.e. at 80% and 120% of VT2.
Interval training test (IT)MDR performed 3 minutes running at 80% of the VT2 speed, for 3 times, with an active recovery (AR) at 60% of VT2 which lasted 3 minutes between repetitions. MDR ran for 3 minutes at 120% of the VT2 speed, for 3 times, with an AR at 60% of VT2 which lasted 3 minutes between repetitions. Before starting both tests athletes performed a 10 min warm-up phase consisting of running at a self-paced speed to fully prepare them for the high peak workloads related to the IT. The two chosen workloads reproduce a common approach to training and testing in athletics and similar protocols have been used in previous works (Crisafulli et al., 2008; Hermansen and Stensvold, 1972; Helgerud et al., 2007). Tests were conducted in a temperature-controlled room (temperature set at 18°C with relative humidity at 50%). All participants were in their best psychological condition: they trained together and their team spirit was high when performing. Moreover, they were motivated to do their best in the trials. During all tests, gas exchange data were collected continuously using an automated breath-by-breath gas analyser (VO2000, Med Graphics, St. Paul, Minnesota). The device weighs about 1.2 kg and includes the metabolic unit, battery pack, harness, chest belt for HR monitoring, face mask, and breathing valve. It was worn on the subject’s chest with a body harness, and did not limit the athlete’s movements. Prior to testing, VO2000 was calibrated according to the manufacturer’s instructions. VO2, VCO2, VE, respiratory exchange ratio (RER) and HR were measured. Achievement of VO2max was considered as the attainment of at least two of the following criteria: 1) a plateau in VO2, despite increasing speed; 2) a respiratory exchange ratio >1.10; and 3) a heart rate ± 10 beats·min1 of predicted HRmax calculated as 220-age (Howley et al., 1995). Oxygen pulse (OP) was calculated as the VO2/HR ratio, which is defined as the quantity of oxygen being consumed by the body for each single cardiac beat and it provides an estimation of stroke volume value (Crisafulli et al., 2007). The oxygen debt (O2debt) as the volume of oxygen consumed above basal values for the first 30 s after each repetition was also assessed (Otsuki et al., 2007). An index of anaerobic glycolysis was obtained by measuring excess CO2 production (CO2excess). This parameter was assessed as follows:
CO2excess = VCO2 (RERrest·VO2)
where RERrest is the respiratory exchange ratio at rest (Anderson and Rhodes, 1989).
The rationale behind the use of CO2excess as an index of lactate (La) accumulation lies in the fact that within the physiological range of pH, lactic acid dissociates and produces H+, which is buffered by –HCO3 and other cell buffers. The amount being buffered by –HCO3 leads to H2CO3 production which in turn dissociates into H2O and CO2 (Beaver et al., 1986).
Statistical analysisData were averaged for the last minute of each recovery period. Repeated one-way ANOVA measurements was applied to determine significance between the various periods of recovery and Newman-Keuls post-hoc was performed when appropriate. Significance was set at p value <0.05.
The evaluation tests were completed by all subjects. The mean maximum speed [± standard deviation (SD)] reached during the indoor incremental tests was 20.0±1.2 km·h1. The Pmean values during IT sessions at 80% and 120% of VT2 were 13.85 ± 0.85 and 15.92 ± 1.02 km·h1 respectively, so they were not matched. HR. During the three active recovery phases (recα, recβ, recγ) after the three repetitions performed at the two speeds, the HR values had progressively increased (Figure 1, left panels). In detail, at 80% of the VT2 speed there was a significant increase between recα and recγ (144 versus 150 beats·min1, p < 0.001), and this trend was particularly evident at the over-threshold intensity where HR rose from 159 to 168 beats·min1 through recα and recγ (p < 0.001). OP. Oxygen pulse showed a declining trend during recovery phases at both speeds (Figure 1 right panels). It was significant between recα and recβ (18.3 versus 17.2 mL·beats1, p < 0.05) and between recα and recγ (18.3 versus 16.4 mL·beats1, p < 0.001) at 80% of the VT2 speed. In tests performed at 120% of the VT2 speed OP was 17.8 mL·beats1 at recα and 16.3 mL·beats1 during recγ (p < 0.05). VO2 and O2debt. The oxygen debt estimation showed a flat trend both in tests carried out at 80% and 120% of the VT2 speed (Figure 2 right panels). Similarly, no difference in oxygen uptake values was observed in the recoveries, except for recα and recγ at 80% of VT2 (Figure 2 left panels). VE. In recoveries after the sub-threshold repetitions, pulmonary ventilation remained stable from recα to recγ. Likewise, no significant differences were found in the series at 120% of VT2 (Figure 3, left panels). CO2excess. Values of this anaerobic capacity index showed no significant differences during the recoveries after running at the two different speeds (Figure 3, middle panels). RER. As confirmation of the protocol setting, the RER values were firmly under the unit value during the AR at 80% and otherwise above the unit value during AR at 120%. Moreover, no differences were found among subsequent ARs in both series at 80 and 120% of VT2 (Figure 3, right panels).
Instrumental measurement of HR is a well-established practice in many sports and in different training situations (Conconi et al., 1982; Leger and Tokmakidis, 1988; Kinnunen and Heikkila, 1998). HR monitoring has therefore long been considered an important means of checking training intensity and energy expenditure in the same way (Achten et al., 2003). The aim of this study was to investigate the use of HR as a parameter to detect the established recovery in interval training sessions, because although extensive literature is available as concerns the use of HR as a parameter to assess energy expenditure and intensity of training, the same cannot be said as concerns the use of HR to monitor recovery. As has long been known, the HR trend during prolonged exercise is characterized by a gradual increase in HR unrelated to the energy expenditure known as cardiac drift (Crisafulli et al., 2006; Ekelund, 1967). According to some authors (Gonzà lez-Alonso et al., 1997; Rowell, 1974), the drift rate appears to be particularly linked to dehydration and the increase in internal temperature (core temperature). Given the duration of our IT tests (28 min) and according to the above-mentioned works, cardiac drift linked to dehydration and hyperthermia is not realistic in our protocol and therefore, from this point of view, during short or middle running splits HR would be considered a reliable index of established recovery. Indeed, a common method in IT sessions in the field is to determine the time of restart between one repetition and the following by monitoring HR, which is taken as a measure of partial or complete restoration of the metabolic starting conditions. However, recent studies have highlighted that acute HR response during intermittent exercise may be markedly disturbed by metabolic conditions (Tschakert and Hofmann, 2013) and, to set the workload peaks and recoveries (Ppeak and Prec), the metabolic markers (as lactate turn points) rather than the %HRmax should be preferred (Tschakert et al., 2015). In line with the aforementioned studies and by programming two different intensities of effort that reproduce two typical ways of training and which are able to elicit two different metabolic patterns, we wanted to investigate the role of another possible cause of cardiac drift, i.e. metabolic acidosis. The main finding of this research was that the cardiac drift was always present during recovery phases after splits at 80% and 120% of VT2 in contrast with the other respiratory and metabolic parameters assessed (VO2, O2debt, CO2excess, RER, VE), whose values were equally re-established in the three recovery phases after repetitions. This fact means that HR and all the other parameters supplied two different indications about the possibility of restart after the repetitions: the first indicated an incomplete recovery while the second did not. It has long been known that an accumulation of La during exercise is associated with a corresponding increase in a rate of VCO2 called CO2excess, which can be considered as an index of La accumulation (Roecker et al., 2000; Tschakert and Hofmann, 2013). In our study therefore, at least in the IT at 120% of VT2, a gradual increase was expected in CO2excess along the three series, but which did not actually occur (Fig. 3, bottom middle panel). Hence, it appears that no correlation between cardiac drift and metabolic acidosis was highlighted in our protocol conditions, contrary to what was expected. To explain this quite surprising result, both duration and intensity of repetitions and the type of recovery should be considered. It is possible that the AR at 60% of VT2 ensured a neutral balance between La accumulation and elimination (Yano, 1987) and in this regard Hermansen and Stensvold (1972) showed that blood La removal was faster when recovery intensity was up to 65% of VO2max i.e., even higher than in our AR. Besides, as recently shown by Tschakert and coll. (2015) in a high intensity IT protocol, in the first bout the metabolic acidosis could lead to an inhibition of glycolysis with a consequent decrease in net blood La production during the subsequent recovery phases. On the contrary, another possible explanation for the absence of CO2excess drift may be that, because of the high training conditions of athletes, a higher number of repetitions or a higher workload is needed to achieve a state of acidosis able to determine a drift of CO2excess. In any case, HR drift during recoveries did not appear linked to the CO2excess. As a consequence of stable CO2excess values, no significant difference in VE was found in the three recovery phases after repetitions. Therefore, HR trends were not even related to pulmonary ventilation. On the basis of the exercise performed, the OP and O2debt trends were also unexpected for us, especially during IT at 120% of VT2. Indeed, during these recovery series a diminished OP was evident because of a stable O2debt together with an increment in HR. Thus, it appears that athletes were able to restart despite an increasing cardiac drift. It is generally accepted that increased HR generates more left ventricular oxygen demand leading to excessive cardiac workload (Otsuki et al., 2007). This fact should explain why it might be opportune to wait for a stable HR during IT. Nevertheless, it does not appear to be the case in our protocol as no increase in VO2 or O2debt was observed in the athletes.
Limitations of the studyConsidering the wide range of variables involved in the actual outcome of recovery status, this study presents some limits. For instance, with blood lactate as a marker of anaerobic muscular work, hydro-electrolytic as well as thermoregulatory conditions were not assessed. In further research, these other metabolic aspects, such as the influence of more prolonged IT on physiological functions, should also be considered. Moreover, as suggested by Tschakert and Hofmann (2013), the mean load between IT protocols with different workload peaks and recoveries should be matched with the use of equation 1, and unfortunately we did not do this. However, in this regard, it is to be noted that the purpose of our study was not primarily to compare the two different metabolic IT sessions, but to test the reliability of the fixed HR values in restart after recoveries in both conditions.
In summary, the use of fixed HR values as a reliable index of established recovery during IT is inaccurate and may be the cause of under-training, even if Ppeak and Prec are assessed by means of a threshold model. The main strength of this study is that it provides a new perspective (as least empirical as possible) on recovery management during training. Another strength is the large amount of variables assessed, i.e. cardiovascular (HR, OP), respiratory (VE) and metabolic (VO2, O2debt, CO2 excess) parameters. Moreover, all these parameters were studied after training at two different speeds, below and above VT2. In our opinion, this research highlights the intriguing possibility of setting the time of restart after repetitions (i.e. at progressively increased HR values) taking into account the cardiac drift phenomenon. Finally, considering the lack of literature on this experimental setting and methodological approach, the present study is in our opinion a valid and initial step in reassessing the use of HR during recovery.
ACKNOWLEDGEMENTS |
We would like to thank Mr. Barry Mark Wheaton for his editorial assistance. We are also grateful to Mr. Nardino Degortes for his technical assistance to the athletes undergoing the running tests. This study was supported by the University of Cagliari, Banco di Sardegna Foundation and Sardinian Regional School of Sport, Italian Olympic Committee.The authors declare no conflicts of interest that are relevant to the content of manuscript. |
|
AUTHOR BIOGRAPHY |
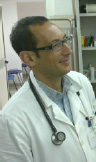 |
Filippo Tocco |
Employment: Researcher, Department of Medical Sciences, School of Sport Medicine, Sport Physiology Lab., University of Cagliari, Italy |
Degree: MD, PhD |
Research interests: Cardiovascular control during exercise in health and disease, diving response, triathlon, athletics. |
E-mail: filippo.tocco@tiscali.it |
|
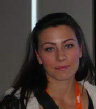 |
Irene Sanna |
Employment: Department of Medical Sciences, School of Sport Medicine, Sport Physiology Lab., University of Cagliari, Italy |
Degree: BSc |
Research interests: Physiological responses and energy expenditure during exercise |
E-mail: |
|
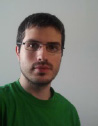 |
Gabriele Mulliri |
Employment: Department of Medical Sciences, School of Sport Medicine, Sport Physiology Lab., University of Cagliari, Italy |
Degree: MD |
Research interests: Ischemic preconditioning |
E-mail: dubbio84@hotmail.com |
|
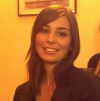 |
Sara Magnani |
Employment: Department of Medical Sciences, School of Sport Medicine, Sport Physiology Lab., University of Cagliari, Italy |
Degree: MD |
Research interests: Stretching, warm ups, fitness training |
E-mail: saretta84.m@gmail.com |
|
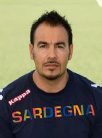 |
Francesco Todde |
Employment: Department of Medical Sciences, School of Sport Medicine, Sport Physiology Lab., University of Cagliari, Italy |
Degree: PhD |
Research interests: Stretching, motor skills, fitness training |
E-mail: francesco_todde@virgilio.it |
|
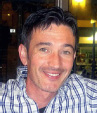 |
Roberto Mura |
Employment: Department of Medical Sciences, School of Sport Medicine, Sport Physiology Lab., University of Cagliari, Italy |
Degree: MD |
Research interests: Cardiovascular phenotype, functional capacity in young soccer players |
E-mail: koenig68@libero.it |
|
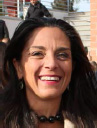 |
Giovanna Ghiani |
Employment: Department of Medical Sciences, School of Sport Medicine, Sport Physiology Lab., University of Cagliari, Italy |
Degree: PhD |
Research interests: Athletics, triathlon, diving response, body composition, chronobiology.
|
E-mail: |
|
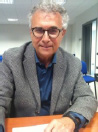 |
Alberto Concu |
Employment: 2C Technologies Inc., Academic Spin-off, University of Cagliari, Italy |
Degree: PhD |
Research interests: Cardiovascular and respiratory control during exercise in health and disease, ischemic preconditioning, muscle metaboreflex, diving response |
E-mail: concu@unica.it |
|
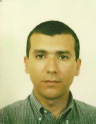 |
Franco Melis |
Employment: Department of Medical Sciences, School of Sport Medicine, Sport Physiology Lab., University of Cagliari, Italy |
Degree: MD, PhD |
Research interests: Cardiovascular control during exercise in elderly adults, muscle metaboreflex, diving response |
E-mail: franco.melis@gmail.com |
|
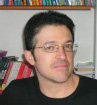 |
Antonio Crisafulli |
Employment: Researcher in the Department of Medical Sciences, School of Sport Medicine, Sport Physiology Lab., University of Cagliari, Italy |
Degree: MD, PhD |
Research interests: Cardiovascular control during exercise in health and disease, ischemic preconditioning, muscle metaboreflex |
E-mail: crisaful@unica.it |
|
|
|
REFERENCES |
 Achten J., Jeukendrup A.E. (2003) Heart rate monitoring, applications and limitations. Sports Medicine 33, 517-538.
|
 Anderson G.S., Rhodes E.C. (1989) A review of blood lactate and ventilatory methods of detecting transition thresholds. Sports Medicine 8, 43-55.
|
 Barnett A (2006) Using Recovery Modalities between Training Sessions in Elite Athletes: Does it Help?. Sports Medicine 36, 781-796.
|
 Beaver L.L., Wasserman K., Whipp B.J. (1986) Bicarbonate buffering of lactic acid generate during exercise. Journal of Applied Physiology 60, 472-478.
|
 Binder R.K., Wonisch M., Corra U., Cohen-Solal A., Vanhees L., Saner H., Schmid J.P. (2008) Methodological approach to the first and second lactate threshold in incremental cardiopulmonary exercise testing. European Journal of Cardiovascular prevention and rehabilitation 15, 726-734.
|
 Bishop P.A., Jones E., Woods A.K. (2008) Recovery From Training: A Brief Review. Journal of Strength and Conditioning Research 22, 1015-1024.
|
 Buchheit M., Laursen P.B. (2013) High-intensity interval training, solutions to the programming puzzle. Part II: anaerobic energy, neuromuscular load and practical applications. Sports Medicine 43, 927-954.
|
 Conconi F., Ferrari M., Ziglio P.G., Droghetti P., and Codeca L. (1982) Determination of the anaerobic threshold by a non invasive test in runners. Journal of Applied Physiology 52, 869-873.
|
 Coyle E.F. (1998) Cardiovascular drift during prolonged exercise and the effects of dehydratation. International Journal of Sports Medicine 19, S121-124.
|
 Crisafulli A., Milia R., Lobina A., Caddeo M., Tocco F., Concu A., Melis F. (2008) Haemodynamic effect of metaboreflex activation in men after running above and below the velocity of the anaerobic threshold. Experimental Physiology 93, 447-457.
|
 Crisafulli A., Piras F., Chiappori P., Vitelli S., Caria M.A., Lobina A., Milia R., Tocco F., Melis F. (2007) Estimating stroke volume from oxygen pulse during exercise. Physiological Measurements 28, 1201-1212.
|
 Crisafulli A., Pittau G., Lorrai L., Carcassi A.M., Cominu M., Tocco F., Melis F., Concu A. (2006) Poor reliability of heart rate monitoring to assess oxygen consumption during field training. International Journal of Sports Medicine 27, 55-59.
|
 Davis J.A., Frank M.H., Whipp B.J., Wasserman K. (1979) Anaerobic threshold alterations caused by endurance training in middle-age men. Journal of Applied Physiology 46, 1039-1046.
|
 Ekelund L.G. (1967) Circulatory and respiratory adaptations during prolonged exercise. Acta Physiologica Scandinavica 70, 5-38.
|
 González-Alonso J., Mora-Rodríguez R., Below P.R., Coyle E.F. (1997) Dehydration markedly impairs cardiovascular function in hyperthermic endurance athletes during exercise. Journal of Applied Physiology 82, 1229-1236.
|
 Goodie J.L., Larkin K.T., Schauss S. (2000) Validation of the Polar heart rate monitor for assessing heart rate during physical and mental stress. Journal Psychophysiology 14, 159-164.
|
 Helgerud J., Høydall K., Wang E., Karlsen T., Berg P., Bjerkaas M., Simonsen T., Helgesen C., Hjorth N., Bach R., Hoff J. (2007) Aerobic high-intensity intervals improve VO2max more than moderate training. Medicine & Science in Sports &Exercise 39, 665-671.
|
 Hermansen L., Stensvold I. (1972) Production and removal of lactate during exercise in man. Acta Physiologica Scandinavica 86, 191-201.
|
 Hiracoba K., Maruyama A., Misaka K. (1996) Prediction of blood lactate accumulation from excess CO2 output during constant exercise. Applied Human Science 15, 205-210.
|
 Howley E.T., Bassett D.R., Welch H.G. (1995) Criteria for maximal oxygen uptake: review and commentary. Medicine & Science in Sports &Exercise 27, 1292-1301.
|
 Kinnunen H, Heikkila I. (1998) The timing accuracy of the Polar Vantage NV heart rate monitor. Journal of Sports Science 16, S107-S110.
|
 Leger L., Tokmakidis S. (1988) Use of the heart rate deflection point to assess the anaerobic threshold. Journal of Applied Physiology 64, 1758-1760.
|
 Meyer T., Faude O., Scharhag J., Urhausen A., Kindermann W. (2004) Is lactic acidosis a cause of exercise induced hyperventilation at the respiratory compensation point. British Journal of Sports Medicine 38, 622-625.
|
 Otsuki T., Maeda S., Iemitsu M., Saito Y., Tanimura Y., Sugawara J., Ajisaka R., Miyauchi T. (2007) Postexercise heart rate recovery accelerates in strength-trained athletes. Medicine & Science in Sports &Exercise 39, 365-370.
|
 Roecker K., Mayer F., Striegel H., Dickhuth H. (2000) Increase characteristics of the cumulated excess-CO2 and the lactate concentration during exercise. International Journal of Sports Medicine 21, 419-423.
|
 Rowell L.B. (1974) Human cardiovascular adjustment to exercise and thermal stress. Physiological Review 54, 75-159.
|
 Tschakert G, Hofmann P. (2013) High-intensity intermittent exercise: methodological and physiological aspects. International Journal of Sports Physiology and Performance 8, 600-610.
|
 Tschakert G., Kroepfl J., Mueller A., Moser O., Groeschl W., Hofmann P. (2015) How to regulate the acute physiological response to “aerobic” high-intensity interval exercise. Journal of Sport Science and Medicine 14, 29-36.
|
 Yano T. (1987) A Theoretical approach to excessive CO2 expiration due to lactate production in exercise. The Japanese Journal of Physiology 37, 937-940.
|
|
|
|
|
|
|