|
|
|
ABSTRACT |
To assess the impact of ‘top-up’ normoxic or hypoxic repeat-sprint training on sea-level repeat-sprint ability, thirty team sport athletes were randomly split into three groups, which were matched in running repeat-sprint ability (RSA), cycling RSA and 20 m shuttle run performance. Two groups then performed 15 maximal cycling repeat-sprint training sessions over 5 weeks, in either normoxia (NORM) or hypoxia (HYP), while a third group acted as a control (CON). In the post-training cycling RSA test, both NORM (13.6%; p = 0.0001, and 8.6%; p = 0.001) and HYP (10.3%; p = 0.007, and 4.7%; p = 0.046) significantly improved overall mean and peak power output, respectively, whereas CON did not change (1.4%; p = 0.528, and -1.1%; p = 0.571, respectively); with only NORM demonstrating a moderate effect for improved mean and peak power output compared to CON. Running RSA demonstrated no significant between group differences; however, the mean sprint times improved significantly from pre- to post-training for CON (1.1%), NORM (1.8%), and HYP (2.3%). Finally, there were no group differences in 20 m shuttle run performance. In conclusion, ‘top-up’ training improved performance in a task-specific activity (i.e. cycling); however, there was no additional benefit of conducting this ‘top-up’ training in hypoxia, since cycle RSA improved similarly in both HYP and NORM conditions. Regardless, the ‘top-up’ training had no significant impact on running RSA, therefore the use of cycle repeat-sprint training should be discouraged for team sport athletes due to limitations in specificity. |
Key words:
Hypoxic training, simulated altitude, top-up training, team sport
|
Key
Points
- ‘Top-up’ repeat-sprint training performed on a cycle ergometer enhances cycle repeat-sprint ability compared to team sport training only in football players.
- The addition of moderate hypoxia to repeat-sprint training provides no additional performance benefits to sea-level repeat-sprint ability or endurance performance than normoxic repeat-sprint training.
- ‘Top-up’ cycling repeat-sprint training provides no significant additional benefit to running RSA or endurance performance than team sport training only, and therefore running based repeat-sprint interventions are recommended for team sport athletes.
|
‘Top-up’ training is a term used to describe training sessions undertaken by athletes in addition to their normal training load, usually comprising 1-3 short duration workouts per week designed to target performance enhancement in a specific aspect of the sport (i.e., repeat-sprint ability). Using ‘top-up’ training in addition to a regular team sport training program first gained scientific attention and support over a decade ago, with soccer players reported to have improved their aerobic capacity by ~11% and in-game running output by ~20% after completing two extra weekly aerobic conditioning sessions over 8 weeks (Helgerud et al., 2001). Since then, methods of ‘top-up’ training in team sports have evolved to now include specific repeat-sprint training, which improves repeat-sprint ability (RSA) (Bravo et al., 2008; Hunter et al., 2011). In a recent review, RSA was defined as ‘the ability to perform repeated short (~3-4 s, 20-30 m) sprints with only brief (~10-30 s) recovery between bouts’, and is described as an integral component of team sport performance (Dawson, 2012). It has also recently become popular to use hypoxic environments for team sport performance enhancement, with recent research (McLean et al., 2013) showing that team sport athletes living at altitude (19 days at ~2130 m) demonstrate similar physiological and performance improvements to those reported in studies using endurance athletes (Robertson et al., 2010). Altitude training has also been proposed to improve physiological determinants of RSA, including phosphocreatine resynthesis rate and VO2max (Bishop and Girard, 2013). Encouragingly, a recent investigation in moderately trained male cyclists found that 4 weeks of cycling repeat-sprint training in hypoxia (RSH) (8 sessions of 3 x 5 x 10 s sprints) significantly improved cycling RSA at sea-level compared to performing a matched training load in normoxia (~40% increase in number of sprints performed vs. ~5% decrease following sea-level training) (Faiss et al., 2013b). Additionally, Puype et al. (2013) investigated much longer sprint durations, finding that 6 weeks of cycling RSH (18 sessions of 4-9 x 30 s sprints) training in healthy males improved muscle phosphofructokinase activity and anaerobic threshold to a greater level than a matched training load performed in normoxia. Regardless, only three known studies have investigated the effect of RSH training in team sport athletes. Galvin et al. (2013) found that 4 weeks of a single set of running RSH (12 sessions of 10 x 6 s sprints on a non-motorised treadmill) in well-trained academy rugby players improved endurance performance, but not RSA compared to the same training performed in normoxia. Brocherie et al. (2015b) recently found that the addition of hypoxia to repeat-sprint training in highly trained youth footballers improved repeated agility performance compared to normoxic training (4.4% vs. 2.0%, respectively) but not RSA. Conversely, Brocherie et al. (2015a) added 6 sessions of RSH (4 x 5 x 5 s overground running sprints) to a live-high / train-low protocol and found that performance in an 8 x 20 m RSA test was significantly enhanced compared to living in altitude and performing the same training in normoxia (3.7 vs. 1.9%, respectively). In support of this multi-set training approach, Goods et al. (2014) demonstrated that oxygen saturation (measured in capillary blood) in team sport athletes performing multiple sets of RSH continued to decrease after the first set of sprints, indicating a more powerful hypoxic stimulus after the first set, which may further enhance the peripheral adaptations expected following RSH, as proposed by Bishop and Girard (2013). Therefore, this study aimed to assess the impact of 5 weeks of multi-set cycling RSH ‘top-up’ training on sea-level cycling RSA, running RSA and endurance performance. Cycling was selected as a non-weight bearing training mode, which is an established method of ‘top-up’ training in elite Australian football during the preparatory phase (Buchheit et al., 2013), intended to increase internal training load without unduly increasing running loads. Whether a cycling protocol can improve sports specific running RSA and running endurance performance was therefore also of interest. It was hypothesised that ‘top-up’ RSH training would provide greater sea-level performance benefits for all outcomes than the same ‘top-up’ training performed in normoxia.
SampleThirty semi-elite male Australian football athletes from one Western Australian football club were recruited for this investigation; mean [95% Confidence Intervals (CI)] age 20.3 [19.7, 20.9] y, stature 1.84 [1.81, 1.86] m, body mass 81.7 [78.7, 84.7] kg. Two participants failed to complete the study due to injuries unrelated to the ‘top-up’ training intervention; one each from the control and hypoxic intervention groups. Prior to commencement, all participants provided written informed consent. Ethical approval was granted by the Human Research Ethics Committee of the University of Western Australia (RA/4/1/5703).
Research designA single blind, randomised control trial design, incorporating 5 weeks of cycling repeat-sprint ‘top-up’ training, in either a hypoxic or normoxic environment, was used to assess the effects on RSA at sea-level. Participants first completed a cycling RSA test, a running RSA test and a 20 m shuttle run test within 7 d, in a randomised order. All participants were instructed to avoid caffeine intake on the day of each test and to keep food intake consistent before each test. Subsequently, participants were evenly allocated into a control (CON), normoxic training (NORM), or hypoxic training (HYP) group via simple randomisation. There were no significant performance differences between groups for any test prior to commencing training. Subsequently, the NORM and HYP groups trained three times per week for 5 weeks in an environmental chamber, performing cycling repeat-sprint training at either sea-level (NORM) or 3000 m of simulated altitude (HYP). Training was planned in a progressive overload manner as a ‘top-up’ to the regular team sport training. Both groups were blinded to their treatment throughout the trial. The CON group continued to train as usual near sea-level (the altitude of Perth, Western Australia is ~30 m), with no ‘top-up’ sessions completed. Regular team sport training was completed together by all groups, and comprised two 60 min sports specific training sessions per week (small sided games, skill and strategy drills), two 45 min strength training sessions, one circuit-based conditioning training session (15 min each of boxing, aerobic cycling intervals and gym circuit) and one 30 min interval running session performed on sand. As the intervention was performed in the pre-competition phase, no matches were played throughout the training period. After completing the 5 weeks of training, all performance tests were repeated to assess the effect of ‘top-up’ training alone, and ‘top-up’ training in hypoxia on performance.
Running Repeat-Sprint Ability TestThis test was performed indoors on a sprung wooden floor. Participants were fitted with a heart rate strap (T31, Polar Electro, Finland), and watch receiver (F1+, Polar Electro, Finland), before they commenced a standardised warm-up, comprising a 2 min jog followed by 3 min of running drills (e.g. high knees, heel flicks) and finally 2 x 20 m submaximal sprints on the test track as a familiarisation. A post-warm-up capillary blood sample was then taken for analysis of blood lactic acid concentration before the test commenced. The running RSA test comprised 3 sets of 6 x 20 m maximal sprints departing every 25 s, each performed from a standing start. Standardised verbal commands were used during each sprint, with times recorded via electronic timing gates (Swift Speed Light Timing System, Swift Performance Equipment, Australia). Upon completing each sprint, participants jogged back to the starting line to commence the next sprint from the common starting end. This test has been used previously (Sim et al., 2009), with excellent test-retest reliability (typical error and coefficient of variance of 0.06 s and 1.8%, respectively, for one set of sprints, and 0.19 s and 1.1% for best 20 m sprint time). Each set was followed by 3 min of passive (standing) and low-intensity (walking) recovery, which included the collection of heart rate, self-rated perceived exertion (Borg’s 6-20 scale; Borg, 1982), and blood lactate following the final sprint. During this time, participants drank water ad libitum from a 750 mL water bottle. Total test duration here was 12.5 min.
Cycling Repeat-Sprint Ability TestA custom made front access cycle ergometer (School of Sport Science, Exercise and Health; University of Western Australia), calibrated prior to use (NATA Ergometer Calibration Rig, Biomed Electronic Services, Australia) was used for the cycling RSA test. Prior to the test, feet were strapped into the pedals and a standardised warm-up comprising 3 min of cycling at 150 W, followed by 3 x 4 s submaximal sprints to familiarise participants with the test protocol was completed. After the warm-up, blood lactate was analysed, and the test commenced 2 min later. The cycling RSA test comprised 3 sets of 6 x 4 s maximal sprints departing every 25 s, performed from a stationary start with the dominant leg forward and parallel to the ground. Standardised verbal commands were used during each sprint. Mean power output and peak power output were recorded for each sprint (Cyclemax, The University of Western Australia, Australia), but not for the subsequent recovery period, which comprised 15 s pedalling at a self-selected intensity followed by 6 s to re-assume the stationary starting position. This protocol was designed to replicate the demands of the running RSA test. Each set was followed by 3 min of self-selected low intensity cycling, where heart rate, blood lactate and perceived exertion were recorded following the final sprint. During this time, participants drank water ad libitum from a 750 mL water bottle. Total test duration here was also 12.5 min.
20 m Shuttle Run TestThis test was also performed indoors on a sprung wooden floor. A standardised warm-up comprising a 2 min jog followed by 3 min of running drills (e.g. high knees, heel flicks) was used pre-test. The shuttle run test was conducted in accordance to the previously established and accepted methods of Leger et al. (1988).
Training interventionAll ‘top-up’ training sessions were performed in the same environmental chamber (mean [CI] temperature and humidity 25.3 [24.9, 25.7] °C and 51 [46.6, 55.4] %, respectively). Simulated altitude in the 40 m3 environmental chamber was achieved through nitrogen injection (flow rate 270 L.min-1, b-Cat BV S879, VPSA S325 V16, Van Amerongen, Biezenwei 6, The Netherlands), creating a normobaric hypoxic environment. The percentage of inspired oxygen for sea-level and 3000 m was 20.9% and 14.5%, respectively. ‘Top-up’ training was performed in groups of 3-5 participants, each using an identical cycle ergometer (M3+ Indoor Cycle, Keiser, USA). Standardised verbal instructions were given throughout each training session. The first session comprised 3 sets of 7 x 5 s maximal sprints (1 repetition and 1 s greater than the cycling RSA test, to overreach testing requirements), with variable recovery times of between 15 s and 35 s between sprints and 3 min of self-selected light active recovery between sets. These training sessions were meant to replicate the duration of high intensity efforts in Australian football, which do not exceed 6 s (Dawson et al. 2004; Gray and Jenkins, 2010), but to exceed the typical repeated sprint repetitions of games, through the addition of a greater than usual number of sprints in a cluster. This approach was taken in respect of the importance of RSA to team sport performance (Gray and Jenkins, 2010). Resistance during sprint (18-20) and recovery (8-10) periods was set to a specified gear range. Progressive overload was applied during the 5 week intervention period by adding 1 sprint to each set per week.
Capillary blood samplesFingertip capillary blood samples (first drop discarded) were taken during the 1st, 8th and 15th training efforts following the warm-up and immediately after each set of repeated sprints to aid in the estimate of training intensity. A 95 µL sample was collected into a heparinised glass capillary tube (Clinitubes, Radiometer Medical ApS, Denmark) and analysed for blood lactate and oxygen saturation (ABL 735, Radiometer Copenhagen, Denmark). During the cycling and running RSA tests, earlobe capillary blood samples were taken following the warm-up and each set of repeated sprints, then immediately analysed for blood lactate (Lactate Pro, Arkray Global Business Inc., Japan).
Perception of training environmentAfter the last post-training performance test, NORM and HYP participants were verbally asked to nominate in which environment they thought they had trained.
Statistical analysisAll data are presented as mean [95% CI]. A between subjects repeated measures ANOVA was used to identify interaction effects between training groups and performance using SPSS analysis software (version 21.0, IBM, USA). Significance was accepted at p≤0.05. Where significant interactions were found, one-way ANOVAs using LSD post-hoc tests were used to clarify any between group differences. Paired samples t-tests were also used to establish within group differences from pre- to post-training. Additionally, changes in performance data between groups were analysed using Cohen’s effect sizes (d), where the following descriptors were used; 0-0.2 (trivial); 0.2-0.6 (small); 0.6-1.2 (moderate); >1.2 (large) (Cohen, 1988). Mean values and Cohen’s effect sizes were both reported with 95% CI to ascertain the certainty with which the results occurred. The smallest worthwhile change in performance variables was deemed to be 0.2*standard deviation (all groups combined at baseline).
Training responsesMean blood lactate concentrations were not different during training sessions (NORM: 7.3 [6.5, 8.1] mmol.L-1 vs HYP: 7.9 [7.0, 8.8] mmol.L-1; p = 0.60), but blood oxygen saturation values were significantly higher for NORM (97.6 [97.4, 97.8] %) than for HYP (89.3 [89.0, 89.6] %; p = 0.001).
Perceptual responsesSix participants from both NORM and HYP groups nominated that they were training in hypoxia, resulting in a 40% correct group assessment for NORM and 67% for HYP.
Cycling repeat-sprint abilityNo significant differences existed between groups at baseline (Figure 1). Following training, mean power output for NORM improved significantly compared to CON in set 2 (p = 0.040; d = 1.17 [0.56,1.77]), with no other significant between group differences found. Mean power output improved significantly in sets one, two, three, and overall in both NORM (p = 0.0001, p = 0.0001, and p = 0.001) and HYP (p = 0.006, p = 0.023, and p = 0.007), whereas CON showed no change. The smallest worthwhile change in mean power output during the test was 25 W, with CON, NORM, and HYP improving by 14 (1.4%), 125 (13.6%), and 88 (10.3%) W respectively. This indicates worthwhile improvement occurred only for NORM and HYP, which was supported by a moderate effect (d = 0.91 [0.39, 1.42]) for a greater improvement in NORM compared to CON; but only a small effect (d = 0.59 [0.04, 1.15]) for a greater improvement in HYP compared to CON. For peak power output, significant improvement for NORM occurred in all three sets (p = 0.002, p = 0.001, and p = 0.0001, respectively), and for HYP improvements in sets 1 (p = 0.028), and 3 (p = 0.041) were noted, with no changes in CON. Significant improvements to peak power output in sets 2 and 3 were found for NORM compared to CON (p = 0.050; d = 0.94 [0.48, 1.42], and p = 0.040; d = 0.94 [0.44, 1.45], respectively), with no other significant between group differences recorded. The smallest worthwhile change in peak power output during the test was 26 W, with CON declining in peak power output by 11 (-1.1%) W, while NORM and HYP improved by 96 (8.6%), and 47 (4.7%) W, respectively. This indicates worthwhile improvement occurred only for NORM and HYP, which was supported by a moderate effect (d = 0.81 [0.39, 1.22] for improved peak power output in NORM compared to CON; but only a small effect (d = 0.49 [0.00, 0.99]) for improvement in HYP compared to CON. After training, a significant time effect across groups existed for a lower heart rate after every set, and for a higher perceived exertion after sets 1 and 2. No significant differences between groups were found for heart rate or perceived exertion (Table 1).
Running repeat-sprint abilityNo significant between group differences existed for running RSA at baseline (Figure 1). Following training, significant time effects for improved (faster) mean sprint times were found in every set in each group. Within groups, mean sprint times significantly improved with training in sets 2, 3 and overall for CON (p = 0.007, p = 0.0001, and p = 0.008, respectively) and NORM (p = 0.002, p = 0.001, and p = 0.006, respectively), and in every set and overall for HYP (p = 0.042, p = 0.004, p = 0.004, and p = 0.005, respectively). The smallest worthwhile change in mean sprint times during the test was 0.02 s, with CON, NORM, and HYP improving by 0.04 (1.1%), 0.06 (1.8%), and 0.08 (2.3%) s respectively. This indicates worthwhile improvement occurred in all groups, with a comparison revealing only a small effect for improved sprint times in NORM (d = 0.21 [-0.15, 0.57] and HYP (d = 0.36 [-0.07, 0.78]) compared to CON. After training, a significant time effect was found for lower blood lactate after set 1 and set 2, as well as lower heart rate following every set. No significant between group differences were found for blood lactate, heart rate or perceived exertion (Table 1).
20 m Shuttle Run TestFollowing training, all three groups significantly increased shuttle number (p < 0.001) with no differences between groups (Figure 1). The smallest worthwhile change in number of shuttles completed during the test was 3 shuttles, with CON, NORM, and HYP improving by 10 (7.1%), 16 (15.4%), and 15 (13.4%) shuttles, respectively. This indicates worthwhile improvement occurred in all groups, with a comparison revealing only a small effect for improved shuttle run performance in NORM (d = 0.41 [-0.01, 0.83] and HYP (d = 0.42 [-0.03, 0.87]) compared to CON.
This study showed that the addition of hypoxia to 5 weeks of ‘top-up’ repeat-sprint training provided no extra benefit to sea-level repeat-sprint performance, compared with the same ‘top-up’ training performed in normoxia. This result concurs with two other team sport RSH training investigations, which found normoxic and hypoxic ‘top-up’ repeat-sprint training equally improved sea-level RSA (Brocherie et al. 2015b; Galvin et al. 2013). In contrast, Brocherie et al. (2015a) recently reported that adding 6 RSH sessions to a live-high / train-low protocol improved RSA by a further 1.7% compared to a group who lived at altitude but performed repeat-sprint training in normoxic conditions (almost doubling the magnitude of improvement). However, while our data showed both groups also significantly improved endurance performance, no substantially greater 20 m shuttle run performance benefit was found following RSH, which is in contrast to the results of Galvin et al. (2013). Since the RSH interventions employed by Galvin et al. (2013) and Brocherie et al. (2015a) (who found endurance and RSA improvements respectively) were both running-based, as compared to the current study where RSH was cycling-based (and no additional performance improvements were noted), the use of non-specific cycling RSH training for team sport athletes is not supported here. However, this needs to be confirmed in an investigation where matched training is performed by two groups who perform either running or cycling ‘top-up’ repeat-sprint training. It is also important to note that cycling ‘top-up’ repeat-sprint training, regardless of environment, demonstrated larger improvements in cycling RSA compared with CON, which reinforces the principle of specificity in training and highlights the importance of team sport athletes performing predominantly running-based training. However, while HYP demonstrated a two-fold improvement in mean sprint time (2.3%) during the running RSA test compared to CON (1.1%), this was very similar to the improvement in NORM (1.8%), and highlights that hypoxia failed to have any impact on performance. Despite demonstrating only a small effect compared to CON, the magnitude of improvements in mean sprint times for running RSA in both HYP and NORM are in agreement with other research investigating the effects of ‘top-up’ running repeat-sprint training (2.1%: Bravo et al. 2008 and 2.6%: Buchheit et al. 2010). These similar results, despite training mode differences, suggest that cycling repeat-sprint training may still improve running RSA. However, since the CON group also demonstrated substantial mean sprint time improvements (albeit approximately half that of NORM and HYP), without significant between groups differences or any moderate-large effects for performance improvement in the training groups, the running RSA improvements noted for HYP and NORM may not be able to be directly attributed to the ‘top-up’ training alone, or potentially at all (given the lack of effect between groups). Additionally, with no greater improvements found for 20 m shuttle run performance following 15 cycling repeat sprint ‘top-up’ training sessions (despite again an approximately two-fold greater improvement in the training groups), running, rather than cycling-based RSH training interventions, might be preferred at this stage for overall team sport performance enhancement. The preferred use of running as a training mode is supported by recent suggestions that RSA enhancements following RSH training may be greater in running compared to cycling (Girard et al. 2013). Previous work from our laboratory also supports this hypothesis, given that we noted a two-fold greater drop in oxygen saturation, and a two-fold greater increase in blood lactate levels (than found here) following a similar repeat-sprint task utilising a running mode (Goods et al. 2014). This greater oxidative and metabolic strain may partially explain why running-based training interventions could result in greater performance outcomes, as suggested by Girard et al. (2013). However, in practice, the impact of 15 additional multi-set, maximal running repeat-sprint sessions on a team sport training program needs to be carefully considered, especially with respect to the total running load imposed on players. To accommodate these ‘top-up’ training sessions, other components, such as skill or tactical workouts (of arguably greater importance for match outcomes) may need to be reduced in order to sensibly manage the overall running load. Although our results suggest a small (~1-3%) improvement in RSA is possible with 5 weeks of ‘top-up’ training, it is suggested that the relative importance of such small changes in one aspect of the sport is quite low when compared to the high cost of implementing hypoxic ‘top-up’ training and modifying regular training loads to accommodate it. Finally, the ~two-fold greater improvement in overall peak power output during the cycling RSA test for NORM as compared to HYP (with NORM, but not HYP, demonstrating a moderate effect for improved peak power output compared to CON) may reflect a potential limitation of RSH training. Performing repeat-sprint training at altitude may even blunt improvements in maximal power, since the training intensity achieved during RSH is lower than at sea-level (Brosnan et al. 2000; Goods et al. 2014). It should also be considered whether the fixed number of sprints performed here in the cycling RSA test prevented any improvements being found later in an uncapped RSA task as reported by Faiss et al. (2013b). These authors suggest that RSH training may not necessarily produce faster mean sprint times, but rather an improved ability to maintain these times for longer. However, this appears an unlikely outcome of our investigation, even if the RSA task had been uncapped, given that higher power outputs were found in NORM compared with HYP in the final two sets. A limitation of the current investigation is that no underlying physiological responses to RSH training were measured. Bishop and Girard (2013) have speculated that an improved phosphocreatine resynthesis rate and increased mitochondrial activity are possible adaptations following RSH training. Such physiological mechanisms are likely vital to repeated sprint efforts, and as such, the influence of ‘top-up’ training on these variables should be analysed in future RSH training studies. Muscle buffer capacity has also been proposed as a positive non-haematological adaptation to hypoxic training (Gore et al. 2007), with suggestions that this may lead to improved RSA (Edge et al. 2006); however, this association remains equivocal (Bishop and Girard, 2013). Additionally, a second limitation to this investigation is that the additional team sport training loads were not able to be quantified; however, the same training was performed as a team by all members of each group, with four or more coaches present at each session to encourage maximal effort by each player. Finally, Girard et al. (2013) proposed using a mobile inflatable hypoxic marquee for RSH training sessions, to accommodate small-sided games or skill-based activities. Such a strategy would allow overground running during RSH training protocols without any additional running loads being added to the overall training program, thereby reducing the use of cycle ergometers in current RSH research. This method was used to great effect by Brocherie et al. (2015a) which remains the only team sport RSH investigation to have found an improved RSA compared to repeat-sprint training in normoxia.
The current investigation found that cycling ‘top-up’ RSH training provided no additional benefit to sea-level RSA as compared to the same training performed in normoxia. However, cycle-based ‘top-up’ repeat-sprint training conducted in both hypoxic and normoxic conditions did substantially improve cycling RSA when compared to regular team sport training where no ‘top-up’ stimulus was applied. Regardless, there was no impact of any ‘top-up’ training on running RSA, and therefore, future research into RSH or regular ‘top-up’ repeat-sprint training in team sport athletes should endeavor to use sports-specific running activity.
ACKNOWLEDGEMENTS |
The authors would like to acknowledge all participants who took part in this study. The authors declare that no conflicts of interest, past or present, existed throughout this investigation. |
|
AUTHOR BIOGRAPHY |
 |
Paul S.R. Goods |
Employment: PhD candidate in the School of Sport Science, Exercise and Health at University of Western Australia., Australia |
Degree: BSc (Hons) |
Research interests: Hypoxic training interventions to enhance team sport performance. |
E-mail: paul.goods@research.uwa.edu.au |
|
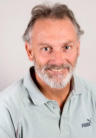 |
Brian Dawson |
Employment: Professor of sport and exercise physiology at the University of Western Australia,, Australia |
Degree: PhD University of Western Australia, Australia |
Research interests: The physiology of intermittent high intensity exercise in the football codes and other team sports. |
E-mail: brian.dawson@uwa.edu.au |
|
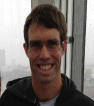 |
Grant J. Landers |
Employment: Lecturer of the School of Sport Science, Exercise and Health at The University of Western Australia |
Degree: PhD |
Research interests: Understanding the body’s physiological response to exercise in extreme environments, recovery from high intensity exercise and anthropometric parameters that influence performance. |
E-mail: grant.landers@uwa.edu.au |
|
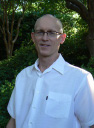 |
Christopher J. Gore |
Employment: Prof. and the Head of the Department of physiology, Australian Institute of Sport, Canberra, Australia. |
Degree: PhD |
Research interests: Altitude training, anti-doping |
E-mail: chris.gore@ausport.gov.au |
|
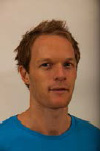 |
Peter Peeling |
Employment: Senior Lecturer at the School of Sport Science, Exercise and Health at the University of Western Australia. |
Degree: PhD |
Research interests: The influence of exercise on iron regulation in athletes. Additionally, Peter is also interested in training-based interventions to enhance athlete performance. |
E-mail: peter.peeling@uwa.edu.au |
|
|
|
REFERENCES |
 Bishop D.J., Girard O. (2013) Determinants of team-sport performance: implications for altitude training by team-sport athletes. British Journal of Sports Medicine 47, 17-21.
|
 Borg G.A.V. (1982) Psychophysical bases of perceived exertion. Medicine and Science in Sports and Exercise 14, 377-381.
|
 Bravo D.F., Impellizzeri F.M., Rampinini E., Castagna C., Bishop D., Wisloff U. (2008) Sprint vs. interval training in football. International Journal of Sports Medicine 29, 668-674.
|
 Brocherie F., Girard O., Faiss R., Millet G.P. (2015b) High-intensity intermittent training in hypoxia: a double-blinded, placebo-controlled field study in youth football players. Journal of Strength and Conditioning Research 29, 226-237.
|
 Brocherie F., Millet G.P., Hauser A., Steiner T., Rysman J., Wehrlin J.P., Girard O. (2015a) “Live-high-train-low and high” hypoxic training improves team-sport performance. Medicine & Science in Sport & Exercise , -.
|
 Brosnan M.J., Martin D.T., Hahn A.G., Gore C.J., Hawley J.A. (2000) Impaired interval exercise responses in elite female cyclists at moderate simulated altitude. Journal of Applied Physiology 89, 1819-1824.
|
 Buchheit M., Mendez-Villanueva A., Delhomel G., Brughelli M., Ahmaidi S. (2010) Improving repeated sprint ability in young elite soccer players: repeated shuttle sprints vs. explosive strength training. Journal of Strength and Conditioning Research 24, 2715-2722.
|
 Buchheit M., Racinais S., Bilsborough J., Hocking J., Mendez-Villanueva A., Bourdon P.C., Coutts A.J. (2013) Adding heat to the live-high train-low altitude model: a practical insight from professional football. British Journal of Sports Medicine 47, i59-i69.
|
 Cohen J (1988) Statistical power analysis for the behavioural sciences. L.Erlbaum Associates.
|
 Dawson B. (2012) Repeated-sprint ability: where are we?. International Journal of Sports Physiology and Performance 7, 285-289.
|
 Dawson B., Hopkinson R., Appleby B., Stewart G., Roberts C. (2004) Player movement patterns and game activities in the Australian Football League. Journal of Science and Medicine in Sport 7, 278-291.
|
 Edge J., Bishop D., Hill-Haas S., Dawson B., Goodman C. (2006) Comparison of muscle buffer capacity and repeated-sprint ability of untrained, endurance-trained and team-sport athletes. European Journal of Applied Physiology 96, 225-234.
|
 Faiss R., Girard O., Millet G.P. (2013a) Advancing hypoxic training in team sports: from intermittent hypoxic training to repeated sprint training in hypoxia. British Journal of Sports Medicine 47, 45-50.
|
 Faiss R., Leger B., Vesin J.M., Fournier P.E., Eggel Y., Deriaz O., Millet G.P. (2013b) Significant molecular and systemic adaptations after repeated sprint training in hypoxia. Plos One 8, -.
|
 Galvin H.M., Cooke K., Sumners D.P., Mileva K.N., Bowtell J.L. (2013) Repeated sprint training in normobaric hypoxia. British Journal of Sports Medicine 47, 74-79.
|
 Girard O., Brocherie F., Millet G.P. (2013) On the use of mobile inflatable hypoxic marquees for sport-specific altitude training in team sports. British Journal of Sports Medicine 47, i121-123.
|
 Goods P.S.R., Dawson B.T., Landers G.J., Gore C.J., Peeling P. (2014) Effect of different simulated altitudes on repeat sprint performance in team sport athletes. International Journal of Sports Physiology and Performance 9, 857-862.
|
 Gore C.J., Clark S.A., Saunders P.U. (2007) Nonhematological mechanisms of improved sea-level performance after hypoxic exposure. Medicine and Science in Sports and Exercise 39, 1600-1609.
|
 Gray A.J., Jenkins D.G. (2010) Match analysis and the physiological demands of Australian football. Sports Medicine 40, 347-360.
|
 Helgerud J., Engen L. C., Wisloff U., Hoff J. (2001) Aerobic endurance training improves soccer performance. Medicine and Science in Sports and Exercise 33, 1925-1931.
|
 Hunter J.R., O’Brien B.J., Mooney M.G., Berry J., Young W.B., Down N. (2011) Repeated sprint training improves intermittent peak running speed in team-sport athletes. Journal of Strength and Conditioning Research 25, 1318-1325.
|
 Leger L.A., Mercier D., Gadoury C., Lambert J. (1988) The multistage 20 metre shuttle run test for aerobic fitness. Journal of Sports Sciences 6, 93-101.
|
 McLean B.D., Buttifant D., Gore C.J., White K., Liess C., Kemp J. (2013) Physiological and performance responses to a preseason altitude-training camp in elite team-sport athletes. International Journal of Sports Physiology and Performance 8, 391-399.
|
 Puype J., Van Proeyen K., Raymackers J. M., Deldicque L., Hespel P. (2013) Sprint interval training in hypoxia stimulates glycolytic enzyme activity. Medicine and Science in Sports and Exercise 45, 2166-2174.
|
 Robertson E.Y., Saunders P.U., Pyne D.B., Gore C.J., Anson J.M. (2010) Effectiveness of intermittent training in hypoxia combined with live high/train low. European Journal of Applied Physiology 110, 379-387.
|
|
|
|
|
|
|