|
|
|
ABSTRACT |
The purpose of this study was to compare the effects of running versus cycling training on sprint and endurance capacity in inline speed skating. Sixteen elite athletes (8 male, 8 female, 24 ± 8 yrs) were randomly assigned into 2 training groups performing either 2 session per week of treadmill running or ergometer cycling in addition to 3 skating specific sessions (technique, plyometrics, parkour) for 8 weeks. Training intensity was determined within non-specific (cycling or running) and effects on specific endurance capacity within a specific incremental exercise test. Before and after the intervention all athletes performed a specific (300m) and one non-specific (30s cycling or 200m running) all-out sprint test according to the group affiliation. To determine the accumulation of blood lactate (BLa) and glucose (BGL) 20 µl arterialized blood was drawn at rest, as well as in 1 min intervals for 10 min after the sprint test. The sport-specific peak oxygen uptake (VO2 peak) was significantly increased (+17%; p = 0.01) in both groups and highly correlated with the sprint performance (r = -0.71). BLa values decreased significantly (-18%, p = 0.02) after the specific sprint test from pre to post-testing without any group effect. However, BGL values only showed a significant decrease (-2%, p = 0.04) in the running group. The close relationship between aerobic capacity and sprint performance in inline speed skating highlights the positive effects of endurance training. Although both training programs were equally effective in improving endurance and sprint capacities, the metabolic results indicate a faster recovery after high intensity efforts for all athletes, as well as a higher reliance on the fat metabolism for athletes who trained in the running group. |
Key words:
Aerobic metabolism, blood glucose concentration, all-out sprint test
|
Key
Points
- In addition to a highly developed aerobic performance inline speed skaters also require a highly trained anaerobic capacity to be effective in the sprint sections such as the mass start, tactical attacks and finish line sprint.
- An 8-week low-intensity endurance training program of either cycling or running training combined with additional routine training improves classical aerobic characteristics (17% increase of VO2 peak), as well as values for acceleration and speed.
- Athletes who trained in the running group demonstrated a higher reliance on the fat metabolism in the sport-specific post-testing.
- The significant reduction in anaerobic ATP turnover during repeated sprints appears to be partially compensated by an increase in VO2 in subsequent sprint. The results revealed a close relationship between the aerobic capacity and sprint performance in inline speed skating.
|
Inline speed skating (ISS) is a mass start endurance sport. Distances in competition are conducted on track or road circuits and range from 200 m to a marathon distance (42.195 km), with completion times between 16 s and 75 min. Due to the tactical strategy (attacks, drafting) and the course (hills, curves, ground) the racing speed fluctuates constantly. In order to compete, inline speed skaters require speed and power (explosive and sustained) combined with a high aerobic and anaerobic capacity (Stangier et al., 2015c). While a highly developed aerobic performance level enables the athletes to sustain a high speed (40-50 km·h-1) in a metabolically economic way (Jones and Carter, 2000) and ensures a fast recovery between the high intensive efforts, a highly trained anaerobic capacity is also required to be effective in the sprint sections such as the mass start, tactical attacks and finish line sprint (Stangier et al., 2015c). Generally, a high aerobic capacity is negatively associated with the sprint performance. However, despite the high average speed and several high intensity tactical sprints, cardiorespiratory demands during an ISS marathon race indicate that as much as 65% of the energy requirements are primarily supplied by the aerobic metabolism (Stangier et al., 2015c). Elite junior cross-country skiers showed a strong relationship between oxygen uptake at the ventilatory threshold and sprint performance (Sandbakk et al., 2011). The significant reduction in anaerobic ATP turnover during repeated sprint exercise, appears to be partially compensated by an increase in V͘O2 in subsequent sprints (Gastin, 2001). This outlines the importance of the aerobic system in cross-country skiing (Vesterinen et al., 2009) that is similar to classic competition procedures in ISS and reasons the relevance of low-intensity endurance training for the sprint capacity. As demonstrated by Seiler et al. (2006, 2010), athletes in several endurance sports follow a polarized model of training intensity distribution. Contrary to the threshold-training model where athletes mostly train at intensities corresponding to lactate threshold, the major part of training under the polarized-training model is spent below the lactate threshold intensity or clearly above the threshold intensity (Seiler, 2010). Several studies proved that an approximate 80-to-20 ratio of low-intensity training to high intensity training effects excellent long-term results in endurance sports (Seiler 2010). Thus, the polarized-training model involves an optimal intensity distribution for training of high-performance endurance athletes during the pre-competition preparation period (Seiler and Kjerland, 2006). The resultant established endurance base built from high volumes of training may be an important precondition for tolerating and responding well to a substantial increase in training intensity in the subsequent training period (Seiler, 2010). Since the optimal period to develop a high level of aerobic fitness is the winter preseason, weather conditions make regular sport-specific training difficult. For this reason inline speed skaters perform a cycling or running training program to improve their basic endurance capacity. However, the cross-training effects of a non-specific training program in these exercise modes on the sport-specific sprint capacity of inline speed skaters are unknown. Although skeletal muscles adapt specifically to a given exercise task over a period of time (Millet et al., 2009), muscle fiber recruitment during running exercise does not only train muscle groups that are specific to running only, but also muscle groups that are used for cycling (Withers et al., 1981). This indicates an overlap between running and cycling in muscle use and presumably some “cross-adaptations” in muscle findings (Pierce et al., 1990). It is likely that there is more physiological training transfer from running to cycling than vice versa (Millet et al., 2009). Specificity is highlighted when peripheral (muscular) adaptations occur without significant concomitant central (cardiovascular) adaptations. As V͘O2 max is peripherally limited by mitochondrial and capillary densities, an exercise mode with sufficient muscle mass would lead to central adaptations covering the specificity of V͘O2 max (Lieber et al., 1989). Loy et al. (1993) and Mutton et al. (1993) have demonstrated significantly improved running performance from either a stair climbing exercise or a combined run and cycle training that were generally comparable to run-only training (Loy et al., 1993, Mutton et al., 1993). Suchlike cross-training induced improvements decrease with increasing aerobic capacity (Loy et al., 1995). Although the concept of specificity is superior to the concept of cross-training (Tanaka, 1994) results of several studies support the concept that non-specific, with similar muscle activity may contribute to enhanced specific performance (Foster et al., 1995). Referring to the present study, running is characterized by a diagonal coordinated cyclic movement pattern of arms and legs similar to ISS. Since the hip is completely stretched and most parts of the involved muscles work dynamically, there is no movement related restriction of blood flow or oxygen supply (Millet et al., 2009). In spite of similar hip angles in the basic position of ISS and cycling, the exercise induced blood flow restriction during ISS is larger due to the relatively long duty cycle (50% vs. 33%) with high static proportions (Foster et al., 1999). Moreover, during cycling the legs work mainly in different direction (flexion and extension vs. abduction and adduction) and arm muscles only work static in contrast to the dynamic arm swing in ISS at high speeds. Although the way in which the propulsive force is generated is quite different between these 3 modes of exercise the fundamental differences in the push-off mechanics and degradation of power rely heavily on the same kinetics of the anaerobic and aerobic power production (van Ingen Schenau et al., 1994). The physiological responses to aerobic exercise as well as exercise testing results appear to be comparable between cycling, running and inline skating (Melanson et al., 1996a; 1996b, Martinez et al., 1993, Stangier et al., 2014). While both, cycling and running exercise affect inline speed skaters†² endurance capacity positively (Stangier et al., 2015b), the present study aims to determine quantitative differences of these non-specific exercise modes on the specific sprint capacity of inline speed skaters. As cycling recruits a higher amount of glycolytic muscle fibers compared to running (47.9% vs. 25%) (Gollnick et al., 1972) we hypothesize that the applied cycling training would induce greater adaptations for the sport-specific sprint capacity than running whereas the running training might have greater effects on the sport-specific endurance capacity.
Participants16 male and female elite athletes (national squad) were recruited for the study, stratified for gender and randomly assigned into 2 exercise groups (running = RUN, cycling = CYC) with 4 male and female athletes in each group. All participants had been active in either national or international competitions over the previous 5 years. Table 1 shows the athletes†² physical characteristics of both training groups with no significant differences between groups on the baseline measures. The athletes were asked to report to all tests well-hydrated and to have refrained from alcohol, caffeine and strenuous exercise for at least 24 h prior to testing. To minimize any possible differences that might arise from changes in food intake, the participants were instructed to maintain a regular diet and to record this for 3 days prior to the pre-testing. The same diet was then adhered on the 3 days before the post-testing. Prior to commencing, the study details were explained to the athletes and their written consent to participate was obtained. Additional parental consent was obtained for those under 18 years. The study received approval from the Institutional Review Board in the spirit of the Helsinki Declaration and according to international standards (Harriss and Atkinson, 2013).
General testing proceduresThe study was conducted from October to December as a part of the preparation period and involved a pre-test, an 8-week training program and a post-test. Prior to the exercise tests, body height (cm) and mass (kg) were measured and recorded. The testing procedures were conducted 1 week before and immediately after the training period. Each athlete performed an incremental step test in ISS to quantify the effect of the non-specific running or cycling training on the specific performance. Dependent on the group affiliation an additional non-specific incremental step test in cycling or running was conducted to determine the individualized training intensities for the non-specific training program. The sprint tests were followed by a resting period of at least 1 hour to provide enough time for lactate removal until the resting state (Monedero and Donne, 2000). All tests were conducted on 2 consecutive days and in randomized order from pre to post-testing at the same time of day to avoid circadian variance. During the incremental step test, intensity increased continuously until the athletes were exhausted or they failed to maintain the pedaling cadence (70-80 rpm) (Neptune and Hull, 1999) or speed. Maximal effort was considered achieved with the attainment of at least 2 of the following criteria: a plateau in oxygen uptake despite increasing work rate; high levels of BLa (8-10 mmol·L-1); a RER above 1.10 and or a HR of ± 10 bpm of age-predicted maximum (220 – age) (Hollmann et al., 2012). During each test, VO2, VE/VO2 and RER were measured breath by breath through a validated (Carter and Jeukendrup, 2002) portable spirograph (Oxycon Mobile, CARE FUSION, Kelberg, Germany). The average of the 3 highest 10 s consecutive measurements were used for analysis. Calibration and ambient air measurement were conducted before each testing session using a precision 3 L syringe (CORTEX, Leipzig, Germany) and calibration gas (15.8% O2, 5% CO2 in N; Praxair, Germany). HR was continuously measured with a HR monitor (S810i, POLAR®, Büttelborn, Germany). To determine blood lactate (BLa) and glucose (BGL) concentrations, arterialized blood samples (20 µl) were extracted from the hyperemic earlobe after each step, immediately placed in a hemolyzing solution and analyzed in our laboratory (BIOSEN C_line, EKF®, London, UK). For this purpose the testing was paused for 1 min after each step to provide time to take the blood sample.
Incremental step testingSpecific and non-specific incremental step tests were conducted to evaluate physiological responses at submaximal and maximum load. The applied protocols of the running, cycling and ISS tests were designed in accordance with established protocols (Heck et al., 1985; Hollmann et al., 1962; Stangier et al., 2014) and to be comparable (same step duration, similar step increment). Previously published results revealed generally comparable physiological responses for these 3 test protocols with a similar amount of exercise steps in each test (Stangier et al., 2014). To ensure a lactate steady state, step durations of 5 min were applied (Bentley et al., 2007; Hollmann et al., 2012). The ISS field test (IFT) (Stangier et al., 2014) was conducted on a 200 m indoor-track. After a low intensity 5 min warm-up the incremental step test started at 24 km·h-1. The speed was then increased stepwise by 2 km·h-1 every 5 min. The running test was conducted on a treadmill (DESMO HP, WOODWAY®, Weil am Rhein, Germany). Subsequent to the low intense self-paced warm-up phase (5 min) the exercise test started with an initial speed of 2.5 m·s-1, which was increased every 5 min by 0.5 m·s-1 (Hollmann et al., 1962). The cycling test was performed on a SRM-ergometer with an eddy brake (SCHOBERER RAD MESSTECHNIK GmbH®, Jülich, Germany). After the warm-up (30 W, 5 min), participants started with the first step at 70 W. Step duration was again 5 min with the intensity increasing by 40 W at each step (Heck et al., 1985).
Sprint testThe specific all-out sprint test was a 300 m track sprint replicating a typical sprint distance in ISS. Each athlete started separately. Time was measured by 6 light barriers and used to compute the corresponding acceleration and speed. The light barriers were positioned at varying distances with a focus on the first and last sprint sections (40m, 80m, 120m, 240m, 280m and 300m). Athletes in the running group performed an all-out 200 m sprint similar to the procedure in the ISS sprint test. Light barriers (TC-System, BROWER TIMING SYSTEMS®, Utah, USA) were positioned at 20m, 60m, 80m, 160m, 180m and 200m). The sprint on the cycle ergometer was a 30 s all-out sprint limited by cadence (60 rpm) (van Ingen Schenau et al., 1988). To determine the accumulation of BLa and BGL 20 µl arterialized blood was drawn at rest, as well as in 1 min intervals for 10 min after each sprint test. The accumulation of BLa was measured to determine the glycolytic rate (Mader, 2003). The determination of BGL concentrations indicate the proportional amount of metabolized carbohydrates within the energy supply (Gastin, 2001).
Training programThe 8-week training program involved 5 sessions per week in 4 different training types, totaling an amount of 7.5 hours. 2 sessions were spent on non-specific endurance training on the cycling ergometer or treadmill (500 kcal per session). The remaining sessions were ISS technique and sprint training in a gym, plyometric strength training and parkour running with imitative jumps each over 90 min. All these sessions were controlled to be identical for both training groups. In order to consider the different amount of propulsive muscle mass and level of efficiency between running and cycling, training intensity was not controlled by metabolic thresholds, rather by individual maximal fat oxidation calculated with stoichiometric equations (Frayn, 1983). The intensity which elicited maximal fat oxidation is highly correlated with the first rise of lactate concentration (Achten and Jeukendrup, 2004). For each participant, breath-by-breath data were used to construct a curve of fat oxidation rate versus exercise intensity, expressed as VO2 . On average, all athletes performed the non-specific endurance training at 52.0 ± 2.5% of VO2peak in the running group and 52.2 ± 2.6% of V͘O2 peak in the cycling group. No significant differences existed in relative training intensities between the groups. After the initial 4 weeks training intensity increased by 10% for both groups to maintain a constant training stimulus. To ensure a comparable training effort for both groups the duration for each participant was adjusted so that energy expenditure was standardized at 500 kcal per session. This method of assigning training intensity was chosen to ensure that all participants trained at their individual maximal fat oxidation rate as this intensity is suggested to improve the aerobic capacity (Coggan et al., 1990). During all training session HR was measured and recorded by a heart rate monitor (S810i, POLAR®, Büttelborn, Germany). Mean and peak HR values and the appropriate RPE-value were documented in a training diary.
Statistical analysisData are presented as mean ± standard deviation and were analyzed using STATISTICA SOFTWARE 7.1 (StaSoft Europe GmbH, Hamburg, Germany). To determine the effects of the training intervention on performance adaptations, the cycling and running group were compared within a 2-way repeated-measure analysis of variance (ANOVA) with “measuring time” serving as the within-group factor and “training group” as the between-group factor. Normal distribution was automatically verified within the ANOVA. Sphericity was proved by the Mauchly†²s test and in case of significant values corrected by the Greenhous-Geisser-Test. To account for the possibility of significant effects in the ANOVA, Fisher†²s protected LSD post-hoc analysis was used to correct p-values accordingly. Statistical significance was accepted at a level of p ≤ 0.05. To examine the relationship between sprint performance in ISS and aerobic characteristics in the post-test status, the Pearson†²s product-moment correlation coefficient test was computed for all participants. Furthermore, a regression analysis was carried out with sprint performance as the dependent variable in simple linear models. The predictor variable was the absolute VO2peak. The reliability of assessments was determined using the technical error of measurement (Pederson and Gore, 1996), and ranged from 1.7% to 3.5% for the analyzed parameters in all tests.
General training resultsOn average, athletes trained between 69 and 75 % of their maximum HR. Similar relative values for HRmean (p = 0.86) and HRpeak (p = 0.77) substantiate that both training groups (cycling and running) performed the ergometer training at comparable training intensities (Table 2). As the comparison of groups showed no effects for gender, all analysis included both genders.
Effects of non-specific endurance exercise on specific sprint performanceThe results showed no significant changes in the accumulation of BLa and BGL. However, all BLa values measured after the specific sprint test decreased significantly (-18%, p = 0.02) from pre to post-testing without any group effect. BGL values only showed a significant decrease (-2%, p = 0.04) in the running group (Figure 1). Mean acceleration (pre and post: 0.6 ± 0.03 m·s-2) and speed (8.9 ± 0.8; 9.3 ± 1.0 m·s-1 p = 0.15) remained unchanged. However, both groups revealed a significant increase of speed in the fourth (240 m: 35.6 ± 3.1, 40.5 ± 4.7 km·h-1; p = 0.002) and fifth (280m: 36.2 ± 3.1, 40.5 ± 4.6 km·h-1; p = 0.01) sprint section, as well as an increase in acceleration (0.4 ± 0.01, 0.5 ± 0.02 m·s-2; p = 0.05 at both sections) indicating a training induced altered pacing strategy. Although the statistical analysis for the sprint time was not significant (29.4 ± 2.6s, 28.8 ± 3.4s; p = 0.27), a reduction of 0.06 s is substantial. Moreover, the sport-specific sprint performance was closely related to VO2peak (p = 0.007; Figure 2).
Effects of non-specific endurance exercise on specific endurance performanceThe analysis of submaximal parameters during the IFT revealed significant effects (p ≤ 0.05) for “measuring time” for all analyzed parameters. Post-hoc analysis showed significant decreases from pre to post measurements for HR, RER and VE/VO2 and a significant increase for VO2, (p ≤ 0.001). Submaximal BLa values remained unchanged. There was no significant group effect from pre to post-testing. The analysis of maximum parameters during the IFT revealed significant effects for “measuring time” for VO2, RER and VE/VO2 (p ≤ 0.05). Post-hoc analysis showed a significant increase for VO2 (p = 0.001), as well as a significant reduction for RER (p = 0.005) and VE/VO2 (p = 0.001). A significant group effect was found in the post-test for maximum HR (p = 0.04) and BLa values (p = 0.05). Both parameters were significantly reduced in the cycling group, and slightly increased in the running group (Table 3).
Non-specific training effects on non-specific sprint and endurance capacityThe analysis of submaximal parameters during the non-specific cycling and running step test revealed significant effects (p ≤ 0.05) for “measuring time” for BLa, RER and VE/VO2, with significant reductions in the post-hoc analysis (p ≤ 0.01). Submaximal HR and VO2 values as well as all analyzed peak values remained unchanged. No significant changes were found from pre to post for the accumulations or level of values for BLa and BGL after both non-specific sprint tests. The running group showed a significant increase in acceleration (1.9 ± 0.41 m·s-2, 2.9 1 ± 0.91 m·s-2; p = 0.003) and speed (22.1 ± 2.4 km·h-1, 25.9 ± 2.9 km·h-1; p = 0.04) in the first sprint section (20m). Sprint performance characteristics in the cycling group remained unchanged. The cycling group revealed a significantly increased mechanical power output from pre (13.3 ± 1.2 W·kg-1) to post (14.7 ± 2.1 W·kg-1; p = 0.004) in the sprint test.
A major finding in this study is the close relationship between the aerobic capacity and sprint performance in ISS. Both non-specific endurance training programs combined with normal training routines improved equally specific aerobic characteristics (17% increase of VO2 peak), as well as values for acceleration and speed at the final sprint part in ISS. This highlights the positive effects of this training type. Moreover the reduction in BLa accumulation indicate a faster recovery after high intensity loads for all athletes, as well as a higher reliance on the fat metabolism for athletes who trained in the running group (Coggan et al., 1990; MacRae et al., 1992). This combination of findings is innovative and may provide important information about the choice and design of future non-specific training in ISS. Sprinting performance relies heavily on fast acceleration at the start of a sprint and the capacity to maintain a high velocity in the phase following the start. In ice speed skating, 50% of the variation in final times of the 500 m sprint during the 1988 Winter Olympics were explained by the differences in initial acceleration (van Ingen Schenau et al., 1994). This highlights the necessity for a short-lasting acceleration phase. Top level sprinters in running, cycling and ice speed skating should be able to produce a mean mechanical power output of approximately 14 to 15 W·kg-1 during a 30 s exercise and approximately 10 to 11 W·kg-1 after 60 s (van Ingen Schenau et al., 1994). Due to the applied methods these values can only be compared with the results of the cycling sprint test in the present study. These 8 athletes revealed a mean mechanical power output of 13.3 ± 1.2 W·kg-1 before and 14.7 ± 2.1 W·kg-1 after the training program within the 30 s all-out sprint. However, a reduction in acceleration time may only be one characteristic in reducing total race time. Arguably the advantage of a fast start is more important than a higher speed at the end of the race. This accounts for the assumption that the remaining energy at the finish would have been wasted as it was not used during the event (van Ingen Schenau et al., 1990, de Koning et al., 1992). However, producing maximal velocities at the beginning is a critical issue as it causes early fatigue and may lead to a massive slowdown later in the race (De Koning and van Ingen Schenau, 2000; Foster and de Koning, 1999). Although a fastest possible acceleration during the starting section is the most important aspect for the sprint performance, an improved time for the closing section (e.g. increased anaerobic capacity) would also be beneficial to reduce the final sprint (Muehlbauer et al., 2010). This advice is supported by the results of the present study, demonstrating that endurance training only improves the closing part of the sprint, as well as the close relationship between aerobic capacity and sprint performance. Such a racing pattern is based on anticipatory regulation of exercise intensity whereby athletes anticipate the work required to complete a given task. This has the advantage to retain an energetic reserve, which protects from early exhaustion and helps to avoid an extensive loss of speed during later race stages (Ulmer, 1996; Foster et al., 2003). While the power output declined by 27% from sprint 1 to 10 the anaerobic ATP utilization decreased by 64% as the glycolytic rate was almost completely inhibited by sprint 10 (Gaitanos et al., 1993). Although an increase in efficiency as exercise continued was suggested as a partial explanation, aerobic metabolism was acknowledged as an important contributor to the energy supply (Nevill et al., 1989). Similar results were found for the post-test sprint performance in this study. Although the sprint time of the specific test remained statistically unchanged (29.4 ± 2.6s, 28.8 ± 3.4s; p = 0.15) after the training program all athletes delivered the similar performance with lower BLa values (Figure 1). The lowered arterial lactate concentrations are affected by increased clearance (Brooks, 2007) and indicate a greater reliance on the aerobic metabolism (Gastin, 2001). Therefore, the applied non-specific training program seems to enable inline speed skaters to recover faster from high intensity sprints. This is particularly relevant in track competitions (men and women: 300m – 1 000m; 23s – 82s) where athletes compete in several heats, as well as during long distance road races (men and women: half and full marathon, 28:56,2 min – 70:43,1 min) after a high intensity attack or climb. During repeated sprint exercise, the significant reduction in anaerobic ATP turnover appears to be partially compensated for by an increase in VO2 in subsequent sprints. This indicates the importance of the aerobic system in determining performance during high intensity exercise (Gastin, 2001). In the current study both, the cycling and the running exercise revealed a significant increase for this parameter (Table 3). This positive effect of the non-specific training on the specific VO2peak (+17%) is consistent with previous investigations reporting a 15% higher VO2peak in moderately trained participants after 9 weeks of either running or inline skating exercise (Melanson et al., 1996a; 1996b). Further evidence in this context is the correlation analysis of the presented data revealing a strong relation between VO2 max and the sprint performance. Another important finding is the significantly reduced BGL values after the specific sprint test for all athletes who trained in the running group. According to the significant group effect, the applied running training might be a greater stimulus for the sport-specific fat metabolism than cycling and effects a higher level of aerobic capacity (Coggan et al., 1990; MacRae et al., 1992). This is possibly related to the hypothesized greater recruitment of oxidative muscle fibers during running compared to cycling exercise (Gollnick et al., 1972) and the greater physiological training transfer from running to cycling than vice versa (Millet et al., 2009). Although skeletal muscle adapts specifically to a given exercise task over a period of time (Millet et al., 2009), muscle fiber recruitment during running exercise trains muscle groups that are specific to running, as well as muscle groups that are used for cycling (Withers et al., 1981) and possibly for ISS. This indicates an overlap in muscle use and presumably some “cross-adaptations” in muscle findings (Pierce et al., 1990). Apart from BGL values there was no significant statistical interaction between the running and cycling training group suggesting that both movement types improved inline speed skaters†² endurance and sprint capacity to a similar extent. The present study is not without limitations. In order to determine possible cross-effects of the additional training sessions (technique, plyometrics, parcour) alone on inline speed skaters†² endurance and sprint capacity an additional control group would have been required. However, the preseason is used to develop a basis in endurance capacity and elite athletes are not willing to change their training routine by omitting training sessions. Although there is a general evidence for positive influences of plyometric training on aerobic characteristics and running economy (Grieco et al., 2012; Ramirez-Campillo et al., 2015; Saunders et al., 2006) in ISS, such a huge increase of V͘O2 peak (+17%) has only been reported for low-intensity training with high volume (Melanson et al., 1996a; 1996b; Stangier et al., 2015a). Moreover, the additional training sessions were matched identically (Table II) between both groups so that these sessions will not have had different influence on the cycling or running training. Furthermore, plyometric training may have a greater influence on sprint than aerobic capacity (Markovic and Mikulic, 2010). Simultaneous explosive-strength and endurance training improved the target time in 5 km time trial in well-trained endurance athletes without changes in their VO2 max. This improvement was due to improved neuromuscular characteristics (i.e. improved intermuscular coordination and stretch-shortening cycle) that were transferred into improved maximal anaerobic speed and running economy (Paavolainen et al., 1999).
The current study demonstrates an 8-week low-intensity endurance training program of either cycling or running training during the preseason combined with additional routine training (technique, parkours, plyometrie), is sufficient to improve classical aerobic characteristics (17% increase of VO2 peak), as well as values for acceleration and speed. Moreover, the results revealed a close relation between the aerobic capacity and sprint performance in ISS highlighting the positive effects of endurance training. Although both training programs were equally effective in improving endurance and sprint capacities, the metabolic results indicate a faster recovery after high intensity efforts for all athletes, as well as a higher reliance on the fat metabolism for athletes who trained in the running group. Moreover the running program would provide the more economical choice as it is less time consuming (~9 min/session). This is relevant in terms of high volume training.
ACKNOWLEDGEMENTS |
The authors would like to thank the athletes and coaches for their participation, commitment and motivation making this project possible. Further thanks go to CARE FUSION Germany, for the loan of the portable Spirograph, Oxycon Mobile.The authors declare that there is no conflict of interest and that the experiments comply with the current laws of Germany, the country in which the study was performed. No external funding was received for this work. |
|
AUTHOR BIOGRAPHY |
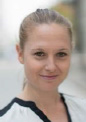 |
Carolin Stangier |
Employment: Institute of Movement and Neurosciences, German Sport University Cologne |
Degree: PhD |
Research interests: Exercise physiology, training and testing |
E-mail: c.stangier@dshs-koeln.de |
|
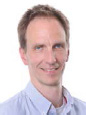 |
Thomas Abel |
Employment: Institute of Movement and Neurosciences, German Sport University Cologne |
Degree: Prof. |
Research interests: Adapted physical activity |
E-mail: abel@dshs-koeln.de |
|
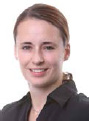 |
Julia Mierau |
Employment: Institute of Movement and Neurosciences, German Sport University Cologne |
Degree: Dr |
Research interests: Exercise physiology, training and testing |
E-mail: j.mierau@dshs-koeln.de |
|
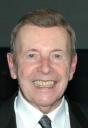 |
Wildor Hollmann |
Employment: Institute of Cardiology and Sports Medicine, German Sport University Cologne |
Degree: Prof. |
Research interests: Preventive medicine, sports medicine, neuroscience |
E-mail: hollmann@dshs-koeln.de |
|
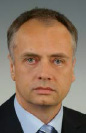 |
Heiko K. Strüder |
Employment: Institute of Movement and Neurosciences, German Sport University Cologne |
Degree: Prof. |
Research interests: Neuroscience, sports medicine |
E-mail: strueder@dshs-koeln.de |
|
|
|
REFERENCES |
 Achten J., Jeukendrup A.E. (2004) Relation between plasma lactate concentration and fat oxidation rates over a wide range of exercise intensities. International Journal of Sports Medicine 25, 32-37.
|
 Bentley D.J., Newell J., Bishop D. (2007) Incremental exercise test design and analysis: implications for performance diagnostics in endurance athletes. Sports Medicine 37, 575-586.
|
 Brooks G.A. (2007) Lactate: link between glycolytic and oxidative metabolism. Sports Medicine 37, 341-343.
|
 Carter J., Jeukendrup A.E. (2002) Validity and reliability of three commercially available breath-by-breath respiratory systems. European Journal of Appiedl Physiology 86, 435-441.
|
 Coggan A.R., Kohrt W.M., Spina R.J., Bier D.M., Holloszy J.O. (1990) Endurance training decreases plasma glucose turnover and oxidation during moderate-intensity exercise in men. Journal of Applied Physiology 68, 990-996.
|
 De Koning J.J., De Groot G., Van Ingen Schenau G. J. (1992) A power equation for the sprint in speed skating. Journal of Biomechanics 25, 573-580.
|
 De Koning J.J., Van Ingen Schenau G.J., Zatsiorsky V.M. (2000) Biomechanics in sport: Performance enhancement and injury prevention. Olympic encyclopaedia of sports medicine. Performance-determining factors in speed skating. Oxford. Wiley-Blackwell Science.
|
 Foster C., De Koning J.J., Gemser H., De Koning J.J., Van Ingen Schenau G.J. (1999) Handbook of competitive speed skating. Physiological perspectives in speed skating. Leeuwarden. Eisma Publishers.
|
 Foster C., De Koning J.J., Hettinga F., Lampen J., La Clair K.L., Dodge C., Bobbert M., Porcari J.P. (2003) Pattern of energy expenditure during simulated competition. Medicine and Science in Sports and Exercise 35, 826-831.
|
 Foster C., Hector L.L., Welsh R., Schrager M., Green M.A., Snyder A.C. (1995) Effects of specific versus cross-training on running performance. European Journal Applied Physiology and Occupational Physiology 70, 367-372.
|
 Foster C., Rundell K.W., Snyder A.C., Stray-Gundersen J., Kemkers G., Thometz N., Broker J., Knapp E. (1999) Evidence for restricted muscle blood flow during speed skating. Medicine and Science in Sports and Exercise 31, 1433-1440.
|
 Frayn K.N. (1983) Calculation of substrate oxidation rates in vivo from gaseous exchange. Journal of Applied Physiology: Respiratory Environmental and Exercise Physiology 55, 628-634.
|
 Gaitanos G.C., Williams C., Boobis L.H., Brooks S. (1993) Human muscle metabolism during intermittent maximal exercise. Jounral of Applied Physiology 75, 712-719.
|
 Gastin P.B. (2001) Energy system interaction and relative contribution during maximal exercise. Sports Medicine 31, 725-741.
|
 Gollnick P.D., Armstrong R.B., Saubert C.W.T., Piehl K., Saltin B. (1972) Enzyme activity and fiber composition in skeletal muscle of untrained and trained men. Journal of Applied Physiology 33, 312-9.
|
 Grieco C.R., Cortes N., Greska E.K., Lucci S., Onate J.A. (2012) Effects of a combined resistance-plyometric training program on muscular strength, running economy, and Vo2peak in division I female soccer players. Journal of Strength and Conditioning Reearchs 26, 2570-2576.
|
 Harriss D.J., Atkinson G. (2013) Ethical standards in sport and exercise science research: 2014 update. International Journal of Sports Medicine 34, 1025-1028.
|
 Heck H., Mader A., Hess G., Mucke S., Muller R., Hollmann W. (1985) Justification of the 4-mmol/l lactate threshold. International Journal of Sports Medicine 6, 117-130.
|
 Hollmann W., Knigge K., Knicker A., Strüder H., Boecker H., Hillman C. H., Scheef L., Strüder H. (2012) Functional Neuroimaging in Exercise and Sport Sciences. Methods for measurement of physical fitness and recommendations in studies on humans. New York, Heidelberg, Dordrecht, London. Springer Science+Business Media.
|
 Hollmann W., Venrath H., Bonnekoh A., Nöcker L. (1962) Investigations on maximum and maximum steady state performance in German elite football players. Sportarzt 6, 172-.
|
 Jones A.M., Carter H. (2000) The effect of endurance training on parameters of aerobic fitness. Sports Medicine 29, 373-386.
|
 Lieber D.C., Lieber R.L., Adams W.C. (1989) Effects of run-training and swim-training at similar absolute intensities on treadmill VO2max. Medicine and Science in Sports and Exercise 21, 655-661.
|
 Loy S.F., Hoffmann J.J., Holland G.J. (1995) Benefits and practical use of cross-training in sports. Sports Medicine 19, 1-8.
|
 Loy S.F., Holland G.J., Mutton D.L., Snow J., Vincent W.J., Hoffmann J.J., Shaw S. (1993) Effects of stair-climbing vs run training on treadmill and track running performance. Medicine and Science in Sports and Exercise 25, 1275-1278.
|
 Macrae H.S., Dennis S.C., Bosch A.N., Noakes T.D. (1992) Effects of training on lactate production and removal during progressive exercise in humans. Journal of Applied Physiology 72, 1649-1656.
|
 Mader A (2003) Glycolysis and oxidative phosphorylation as a function of cytosolic phosphorylation state and power output of the muscle cell. European Journal of Applied Physiology 88, 317-338.
|
 Markovic G., Mikulic P. (2010) Neuro-musculoskeletal and performance adaptations to lower-extremity plyometric training. Sports Medicine 40, 859-895.
|
 Martinez M.L., Ibanez Santos J., Grijalba A., Santesteban M.D., Gorostiaga E.M. (1993) Physiological comparison of roller skating, treadmill running and ergometer cycling. International Journal of Sports Medicine 14, 72-77.
|
 Melanson E.L., Freedson P.S., Jungbluth S. (1996a) Changes in VO2max and maximal treadmill time after 9 wk of running or in-line skate training. Medicine and Science in Sports and Exercise 28, 1422-1426.
|
 Melanson E.L., Freedson P.S., Webb R., Jungbluth S., Kozlowski N. (1996b) Exercise responses to running and in-line skating at self-selected paces. Medicine and Science in Sports and Exercise 28, 247-250.
|
 Millet G.P., Vleck V.E., Bentley D.J. (2009) Physiological differences between cycling and running: lessons from triathletes. Sports Medicine 39, 179-206.
|
 Monedero J., Donne B. (2000) Effect of recovery interventions on lactate removal and subsequent performance. International Journal of Sports Medicine 21, 593-597.
|
 Muehlbauer T., Schindler C., Panzer S. (2010) Pacing and sprint performance in speed skating during a competitive season. International Journal Sports Physiology and Performance 5, 165-176.
|
 Mutton D.L., Loy S.F., Rogers D.M., Holland G.J., Vincent W.J., Heng M. (1993) Effect of run vs combined cycle/run training on VO2max and running performance. Medicine and Science in Sports and Exercise 25, 1393-1397.
|
 Neptune R.R., Hull M.L. (1999) A theoretical analysis of preferred pedaling rate selection in endurance cycling. Journal of Biomechanics 32, 409-415.
|
 Nevill M.E., Boobis L.H., Brooks S., Williams C. (1989) Effect of training on muscle metabolism during treadmill sprinting. Jounral of Applied Physiology 67, 2376-2382.
|
 Paavolainen L., Hakkinen K., Hamalainen I., Nummela A., Rusko H. (1999) Explosive-strength training improves 5-km running time by improving running economy and muscle power. Journal of Applied Physiology 86, 1527-1533.
|
 Pederson D., Gore C., Norton K., Nolds T. (1996) Anthropometrica: A textbook of body measurement for sports and health courses. Anthropometry measurement error. Sydney. UNSW Press.
|
 Pierce E.F., Weltman A., Seip R.L., Snead D. (1990) Effects of training specificity on the lactate threshold and VO2 peak. International Journal of Sports Medicine 11, 267-272.
|
 Ramirez-Campillo R., Vergara-Pedreros M., Henriquez-Olguin C., Martinez-Salazar C., Alvarez C., Nakamura F.Y., De La Fuente C.I., Caniuqueo A., Alonso-Martinez A.M., Izquierdo M. (2015) Effects of plyometric training on maximal-intensity exercise and endurance in male and female soccer players. Journal of Sports Science , 1-7.
|
 Sandbakk O., Welde B., Holmberg H. C. (2011) Endurance training and sprint performance in elite junior cross-country skiers. Journal of Strength and Conditioning Research 25, 1299-1305.
|
 Saunders P.U., Telford R.D., Pyne D.B., Peltola E.M., Cunningham R.B., Gore C.J., Hawley J.A. (2006) Short-term plyometric training improves running economy in highly trained middle and long distance runners. Journal of Strength and Conditioning Research 20, 947-954.
|
 Seiler K.S., Kjerland G.O. (2006) Quantifying training intensity distribution in elite endurance athletes: is there evidence for an "optimal" distribution?. Scandinavian Journal of Medicine and Science in Sports 16, 49-56.
|
 Seiler S (2010) What is best practice for training intensity and duration distribution in endurance athletes?. International Journal of Sports and Physiology Performance 5, 276-291.
|
 Stangier C., Abe T., Hesse C., Claßen S., Mierau J., Struder H. K. (2015a) Abstracts Book of 20th Anual Congress European College of Sports Science. Effects of Cycling vs. Running Training on Endurance Performance in Preparation for Inline Speed Skating. Malmö-Sweden. Book of Abstract.
|
 Stangier C., Abel T., Hesse C., Claen S., Mierau J., Hollmann W., Struder H. K. (2015b) Effects of Cycling vs. Running Training on Endurance Performance in Preparation for Inline Speed Skating. Journal of Strength and Conditioning Research. press , -.
|
 Stangier C., Abel T., Mierau J., Gutmann B., Hollmann W., Strüder H. K. (2014) Comparison of sport-specific and non-specific exercise testing in inline speed skating. Journal of Sports Medicine and Physical Fitness , -.
|
 Stangier C., Abel T., Mierau J., Gutmann B., Hollmann W., Strüder H. K. (2015c) Cardiorespiratory Demands During an Inline Speed Skating Marathon Race - A Case Report. Journal of Sports Medicine and Physical Fitness , -.
|
 Tanaka H (1994) Effects of cross-training. Transfer of training effects on VO2max between cycling, running and swimming. Sports Medicine 18, 330-339.
|
 Ulmer H.V. (1996) Concept of an extracellular regulation of muscular metabolic rate during heavy exercise in humans by psychophysiological feedback. Experientia 52, 416-420.
|
 Van Ingen Schenau G.J., De Boer R.W., Geysel J.S., De Groot G. (1988) Supramaximal test results of male and female speed skaters with particular reference to methodological problems. European Journal of Applied Physiology and Occupational Physiology 57, 6-9.
|
 Van Ingen Schenau G.J., De Koning J.J., De Groot G. (1990) A simulation of speed skating performances based on a power equation. Medicine and Science in Sports and Exercise 22, 718-728.
|
 Van Ingen Schenau G.J., De Koning J.J., De Groot G. (1994) Optimisation of sprinting performance in running, cycling and speed skating. Sports Medicine 17, 259-275.
|
 Vesterinen V., Mikkola J., Nummela A., Hynynen E., Hakkinen K. (2009) Fatigue in a simulated cross-country skiing sprint competition. Journal of Sports Science 27, 1069-1077.
|
 Withers R.T., Sherman W.M., Miller J.M., Costill D.L. (1981) Specificity of the anaerobic threshold in endurance trained cyclists and runners. European Journal of Applied Physiology and Occupational Physiology 47, 93-104.
|
|
|
|
|
|
|