|
|
|
ABSTRACT |
The purpose of the presented study was to compare acute and post-exercise differences in cardiorespiratory, metabolic, cardiac autonomic, inflammatory and muscle damage responses to high-intensity interval exercise (HIIT) between endurance and sprint athletes. The study group consisted of sixteen highly-trained males (age 22.1 ± 2.5 years) participating in endurance (n = 8) or sprint (n = 8) sporting events. All the participants underwent three exercise sessions: short HIIT (work interval duration 30s), long HIIT (3min) and constant load exercise (CE). The exercise interventions were matched for mean power, total time and in case of HIIT interventions also for work-to-relief ratio. The acute cardiorespiratory (HR, V̇O2, RER) and metabolic (lactate) variables as well as the post-exercise changes (up to 3 h) in the heart rate variability, inflammation (interleukin-6, leucocytes) and muscle damage (creatine kinase, myoglobin) were monitored. Endurance athletes performed exercise interventions with moderately (CE) or largely (both HIIT modes) higher mean V̇O2. These differences were trivial/small when V̇O2 was expressed as a percentage of V̇O2max. Moderately to largely lower RER and lactate values were found in endurance athletes. Markers of cardiac autonomic regulation, inflammation and muscle damage did not reveal any considerable differences between endurance and sprint athletes. In conclusions, endurance athletes were able to perform both HIIT formats with increased reliance on aerobic metabolic pathways although exercise intensity was identical in relative terms for all the participants. However, other markers of the acute and early post-exercise physiological response to these HIIT interventions indicated similarities between endurance and sprint athletes. |
Key words:
Intermittent exercise, training mode, heart rate variability, inflammation, muscle damage
|
Key
Points
- The manner in which each training background (endurance vs. sprint) influences the response to HIIT is not well known.
- Despite the identical exercise intensity in relative terms, endurance athletes are able to perform HIIT with increased reliance on aerobic metabolic pathways when compared to sprint athletes.
- The mean O (% O) and HR as well as markers of the cardiac autonomic regulation, systemic inflammation and muscle damage monitored during the early recovery phase did not demonstrate any differences between endurance and sprint trained individuals.
|
High-intensity muscle work is often required under any competitive sport conditions, no matter whether it is an endurance, sprint/power event or a combination (sport games). Since most sports performances last several minutes to hours, both aerobic and anaerobic capabilities in varied proportions are always necessary. Each athlete is unique, however, and can be characterized from a number of points of view, including the capacity to perform mostly aerobic or mostly anaerobic events as well as the ability to successfully repeat and maintain high-intensity exercise for a prolonged period (Spencer et al., 2005). Muscle fibre type distribution, metabolic and cardiorespiratory regulation and aerobic capacity enable endurance athletes to have an advantage in prolonged endurance events, whereas sprint/power athletes are better suited for high-intensity, short-term, and explosive activities (Kenney et al., 2015). This simple classification is challenged, however, when exercise is described as a combination of both a high-intensity workload and prolonged duration. High-intensity interval training (HIIT) is a widely used and effective training method in various sports, including both endurance and sprint/power events (Milanović et al., 2015). HIIT requires an integration of a number of physiological systems. The contributions of ATP-phosphocreatine (PCr) and glycolytic metabolic pathway are necessary for achieving high exercise intensity whereas an oxidative metabolic pathway is crucial for maintaining high exercise intensity as long as possible (Buchheit and Laursen, 2013; Tschakert and Hofmann, 2013). Ufland et al. (2013) have demonstrated that sprinters have a lower mean repeated sprint time, but simultaneously also a lower repeated sprint ability. In addition, innate endowment, training history and a consequent ability to perform endurance or power/speed exercise certainly entails that even identical muscle work can be performed with more aerobic and less anaerobic contribution and vice versa. Therefore, a cross-sectional study could be useful in order to assess the manner in which each training background (endurance vs. sprint) influences the response to HIIT, providing important information to assist coaches in adjusting training programs to athlete-specific metabolic characteristics and developing strategies to improve performance. The response to any stress, including exercise, is complex, highly variable, and involves a myriad of adaptive responses in multiple organ systems (Zierath and Wallberg-Henriksson, 2015). We therefore focused on multiple physiological variables of acute as well as post-exercise response to HIIT. Apart from the description of the acute cardiorespiratory (heart rate, oxygen consumption and carbon dioxide production) and metabolic (lactate) response, post-exercise heart rate variability (HRV) was assessed. HRV is considered a tool for cardiac autonomic regulation assessment which provides information about exercise load and post-exercise recovery (Buchheit, 2014). Biochemical markers of exercise-induced inflammation (interleukin-6, leucocytes) and muscle damage (creatine kinase, myoglobin) were also evaluated (Paulsen et al., 2012). Interleukin-6 (IL-6) has been reported to have pro- as well as anti-inflammatory effects and might play an important role in metabolic and musculoskeletal adaptation to exercise (Pedersen and Febbraio, 2012). The high intensity character of HIIT can potentially lead to muscle fibre impairment which can be manifested by increases in concentration of creatine kinase (CK) and myoglobin in plasma (Paulsen et al., 2012). The direct relationships between IL-6, CK, myoglobin versus exercise intensity and duration have been previously noted (Chen et al., 2007; Cullen et al., 2016). The primary aim of this study was to compare the acute cardiorespiratory and metabolic response to various modes of HIIT between endurance and sprint trained athletes. We assume that endurance trained athletes perform HIIT interventions with lower acute cardiorespiratory and metabolic responses despite a high peak workload intensity, primarily due to their faster oxygen uptake kinetics (Berger and Jones, 2007) and greater maximal muscle oxidative capacity (Dubouchaud et al., 2000) associated with a greater reliance on fat as fuel for the energy supply, more effective acid-base status control (Hawley, 2002) and lactate removal ability (Thomas et al., 2004). Additionally, potential exercise-induced changes in HRV, IL-6, leucocytes, and muscle damage markers may provide unique holistic insight into the question of differences between endurance and sprint type athletes in response to a single bout of HIIT.
ParticipantsSixteen highly-trained males volunteered in this study (Table 1). All the participants were deliberately approached and chosen in order to match the specification of the study subgroups, i.e. regular sport training with the aim of preparing for official national or international competitions in endurance or sprint sport disciplines. Endurance athletes participated in at least one of the following: 5 km run (1 athlete), tower-running (1 athlete), sky/trail-running (3 athletes), triathlon (2 athletes), long-track in-line skating (1 athlete), cross-country skiing (1 athlete). Sprint athletes participated in 100-400 meters track run. None of the participants were clinically diagnosed with any chronic or acute cardiovascular, metabolic, respiratory, immunological or musculoskeletal system disorders. None of the participants used any medication. Prior to the participant's involvement, the local Ethics Committee of the University approved the experimental protocol and the investigation conformed to the principles outlined in the Declaration of Helsinki. All participants were fullyinformed about the study details and provided written informed consent.
Experimental designThe participants visited the laboratory on four separate occasions over a 1-2 week interval. During this time, they firstly performed a maximal incremental treadmill test. They consequently performed a short HIIT, a long HIIT, and one constant load exercise (CE) session matched for mean load and total duration. The order of the exercise sessions was chosen at random. All sessions were performed in the morning and were conducted by the same researchers in a thermally-controlled laboratory room.
Preliminary ProceduresAll the participants were informed about the experimental procedure during the first laboratory visit. They also completed a short questionnaire about physical activity, acute or chronic diseases and the use of dietary supplements/medication. Anthropometric assessment and a body composition analysis then followed (Tanita BC418MA; Japan). In order to determine their maximum aerobic capacity (V̇O2max), the minimal running speed required to elicit V̇O2max (θ½V̇O2max), as well as the first and second ventilatory thresholds (VT1, VT2), participants performed a graded exercise test (GXT) as previously described (Cipryan et al., 2016). Expired air was continuously monitored for an analysis of O2 and CO2 concentrations during the GXT by the use of a breath-by-breath system (ZAN600Ergo; Germany). It was determined that the participants had reached their V̇O2max, when at least two of the following criteria were met: (A) a plateau in the V̇O2 or an increase less than 2.1 mL·kg-1·min-1 despite the increasing running speed, (B) a final respiratory exchange ratio (RER) higher than 1.10; (C) an attainment of 95% of the age-predicted maximal heart rate (HR). The V̇O2max was based on the highest average O2 consumption measured during a 30 s period. Gas-exchange measurements were also used to quantify the first and second ventilatory thresholds (VT1 and VT2) (Hofmann and Tschakert, 2011). VT1 was defined as the first increase in ventilation and equivalent for oxygen consumption (VE/VO2), without an increase of the equivalent for the carbon dioxide production (VE/VCO2). VT2 was defined as the second increase in VE with an increase in both VE/VO2 and VE/VCO2. The final incremental test speed reached at the end of the test (vinc.t.) and at the V̇O2max were calculated (Kohn et al., 2011). Heart rate was measured using a chest belt (Polar Electro; Finland). The maximal countermovement jump height (Haff and Dumke, 2012) and the 30s Bosco test (Bosco et al., 1983) were performed 30-40 min before GXT in order to precisely distinguish the differences between the endurance and sprint study groups.
Exercise interventionThe following visits to the laboratory consisted of interval and continuous exercise interventions. Participants always arrived at the laboratory between 7 and 9 a.m., after a night of fasting (i.e., no breakfast was consumed). Detailed prescriptions for long and short HIIT and CE are shown in Table 2. The 8 min warm-up at 50 % θ½V̇O2max was performed before both HIIT. The exercise interventions were ended by 3 min of walking at 5 km.h-1. All three exercise tests were matched for the total duration and mean power (Tschakert and Hofmann, 2013). Long (3 min) and short intervals (30 s) were identical for work/relief ratio as well as for the relative work and relief intensity. Ventilatory parameters and HR were monitored during the exercise. Blood lactate concentrations obtained from capillary blood samples taken from a finger (20 µL) were measured before exercise, at the end of warm-up (7-8th min), and then after each long (3 min) intervals or in identical time pattern in case of short intervals and constant load exercise (i.e. in 12th, 18th, 24th, and 30th min) (Accutrend Plus, Roche, Germany).
Recovery monitoringThe participants remained resting in the laboratory for 3 h post testing to assess the recovery process. HRV was measured in the supine position before and after the exercise, 1 h, 2 h, and 3 h after the exercise intervention. Blood samples were collected in the sitting position from the antecubital vein before (PRE) and immediately after the exercise (POST), 1 h, and 3 h after the exercise intervention. Fluid and food ingestion after each exercise intervention was standardized. Accordingly, each participant was provided with carbohydrate-rich, low-fat food (plain sponge biscuits 240 g; 75,0 g CHO, 11.0 g protein and 4.9 g fat per 100 g; 390 kcal per 100 g) and 1.5 l of sweet mineral water (21.4 kcal per 100 mL).
HRV analysisThe last 5 min periods of the 10 min supine rest ECG recording data were analysed using VarCor PF8 (Dimea Group Ltd, Czech Republic). This diagnostic system enables a routine short-term HRV evaluation with respect to Task Force (Malik et al., 1996) findings and recommendations. ECG was sampled at 1000 Hz and the accuracy of the measurements was 1 ms. The RR data was visually validated prior to analysis, i.e. assessment for stationary, ectopic, missing data or aberrant beats. Ectopic beats were excluded. Based on the recommendation of Plews et al. (Plews et al., 2013), the vagaly-derived HRV parameter rMSSD (the square root of the mean sum of the squared differences between R-R intervals; ms) was used within this study for post-exercise cardiac autonomic modulation assessment. HRV analysis was limited to rMSSD since it reflects vagal activity (Malik et al., 1996) and has a much greater reliability than other spectral indices (Cipryan, 2016b) particularly during ‘free-running’ ambulatory conditions (Penttila et al., 2001).
Venous blood sampling and blood analysisVenus blood was collected into serum separator tubes. The samples were allowed to clot for 30 min and subsequently centrifuged at 2000 G for 10 min in order to separate the serum. The blood serum was consequently divided into three 1 mL aliquots, which were frozenat-70°C until analysis. In addition, a separate blood sample was also collected to assess the leucocytes concentration. The S-Monovette® system (Sarstedt, Germany) was used for blood sample collection. Blood samples were analysed for high sensitive IL-6 (IL-6), leucocytes, creatine kinase (CK), and myoglobin (Mb). The IL-6 concentrations were measured using a high sensitivity Quantikine ELISA kit (R & D Systems, Minneapolis, MA, USA) on a DSX device (DSX, Dynex Technologies, Chantilly, VA, USA). Leucocytes were measured with a UNICEF DxH 800 Coulter device. CK was measured with an AU 2700 device (Beckman Coulter, Inc., Brea, CA). Myoglobin (Mb) was measured with a Unicel Dxi 800 instrument (Beckman Coulter, Inc., Brea, CA). The analysis of IL-6, leucocytes, CK, and Mb revealed intra-assay coefficients of a variation of 4.4, 3.0, 5.7, and 3.8 %, respectively.
Statistical analysisCollected data were checked to detect outliers and to verify sampling distribution (Shapiro-Wilk test; P < 0.05). Outliers were removed and not included in the statistical analysis. An observed (or log-transformed) value was detected as an outlier if it was less/greater than the lower/upper quartile ± 1.5 times the interquartile range. The data were log-transformed using a natural logarithm if a non-normality or heteroscedasticity was revealed. Since traditional null hypothesis significance testing has been extensively criticised (Nuzzo, 2014; Hopkins and Batterham, 2016; Wasserstein and Lazar, 2016) magnitude based inferences were employed for further statistical analysis. Standardised changes in mean (Effect size, ES) and 90% confidence limits (90 % CL) were calculated for differences between groups. Threshold values for ES statistics were <0.2 (trivial), ≥0.2 (small), ≥0.6 (moderate), ≥1.2 (large), ≥2.0 (very large), ≥4.0 (nearly perfect). The exact probabilities were expressed and the magnitude of the difference was also evaluated qualitatively as follows: 25-75% possibly, 75-95% likely, 95-99.5% very likely, >99.5% most likely. The probability that the true difference was substantial was estimated from the smallest worthwhile change/difference (0.2 x between-individual standard deviation). If the probability of higher or lower differences was >5%, i.e. the confidence interval overlapped the thresholds for substantiveness, then the true difference was deemed unclear (Batterham and Hopkins, 2006; Hopkins et al., 2009). Statistical analyses were performed using statistical spreadsheets (Hopkins, 2006) and IBM SPSS Statistics 23.
Preliminary testingAll the results obtained from GXT, the countermovement jump height test and the 30s Bosco test are presented in Table 3. There were moderate (θ½V̇O2max, vinc.t., vVT1, GXT duration) to large (V̇O2max, vVT2, countermovement height jump) differences between most of the variables in the endurance vs. sprint athlete comparisons. The differences between the study groups in HRmax, VT1, VT2, RPE and Bosco test were unclear.
Cardiorespiratory and lactate responseThe differences between endurance and sprint athletes in the HR response (both expressed in absolute values or as % of individual HRmax) were unclear for all the exercise interventions (Table 4, Figure 1). Endurance athletes performed short HIIT, long HIIT and CE with largely, very largely, and moderately, respectively, higher mean V̇O2. These differences were trivial to small when V̇O2 was expressed as a function of V̇O2max (Table 4, Figure 2). Moderately to largely lower RER and lower lactate values were found in endurance athletes (Table 4, Figure 3 and 4">4).
Post-exercise HRVThe ln rMSSD values decreased immediately after both HIIT with the most pronounced changes in HIIT with long intervals. Ln rMSSD subsequently returned to the baseline values 1 h after the exercise cessation. The differences between endurance and sprint athletes in response to all the exercise interventions were not substantial (Figure 5A).
Interleukin-6 (IL-6) and leucocytesThe post-exercise concentrations in IL-6 and leucocytes increased in all exercise interventions with a subsequent drop in 1h and 3h samples. The differences between endurance and sprint athletes in response to the exercise interventions were not substantial. Small differences in response to the exercise interventions were observed in 1h vs. PRE comparisons in the short HIIT for IL-6 (ES ± 90% CL; -0.53 ± 0.67) as well as with leucocytes (0.52 ± 0.70). A moderate difference in the response between groups was found in the POST vs. PRE comparison (0.84 ± 0.60) for leucocytes changes in the long HIIT (Figure 5B-C).
Creatine kinase (CK) and myoglobinAll the differences in the CK response to the exercise Intervention for the endurance vs. sprint athletes were trivial. Small to moderate differences were observed in the myoglobin response to the short HIIT (-0.51 ± 0.44 and -0.95 ± 1.02 for POST vs. PRE and 1h vs. PRE, respectively), long HIIT (-1.02 ± 1.22 for 3h vs. PRE) and CE (0.56 ± 0.57 for 1h vs. PRE) (Figure 5D-E).
Creatine kinase (CK) and myoglobinAll the differences in the CK response to the exercise intervention for the endurance vs. sprint athletes were trivial. Small to moderate differences were observed in the myoglobin response to the short HIIT (-0.51 ± 0.44 and -0.95 ± 1.02 for POST vs. PRE and 1h vs. PRE, respectively), long HIIT (-1.02 ± 1.22 for 3h vs. PRE) and CE (0.56 ± 0.57 for 1h vs. PRE) (Figure 5D-E).
The major overall finding of this study was that endurance and sprint athletes differed only in the metabolic manner in which they performed a single bout of HIIT whereas the acute cardiorespiratory and post-exercise autonomic and biochemical variables did not manifest any considerable differences in response to the various forms of HIIT. The sprint athletes performed HIIT with moderately to largely higher RER and moderately higher lactate values than endurance athletes even though exercise intensity was individually adjusted at an identical relative intensity (% θ½V̇O2max).
Acute cardiorespiratory responseFive HIIT formats have recently been introduced (Buchheit and Laursen, 2013). Two of them, HIIT with long intervals (work duration >2-3 min) and HIIT with short intervals (work duration ≥15 s), were employed within this study. Intervals with long work durations (long HIIT) induce a higher anaerobic glycolytic energy contribution and higher neuromuscular load than intervals with short work duration (short HIIT) (Buchheit and Laursen, 2013). HIIT can also be classified as aerobic or anaerobic, based on the presence or lack of a lactate steady state (Tschakert and Hofmann, 2013). The long HIIT clearly generated a higher cardiovascular response (higher peak HR values and HR oscillation) whereas the mean HR was similar for both HIIT formats. As was previously shown by Tschakert et al. (Tschakert et al., 2015), this is the expected finding of the presented study since the mean load and exercise duration were identical for both HIIT formats. Most importantly, there were no substantial differences between endurance and sprint athletes in the HR response to both HIIT formats and CE. A substantially more extensive V̇O2 response was elicited during the long HIIT with similar high V̇O2 peaks (% V̇O2max) exceeding VT2 in both endurance and sprint athletes (Figure 2). This greater V̇O2 response was in all probability associated with the development of a V̇O2 slow component (Åstrand et al., 1960) as well as with the exercise intensity prescribed at 100 % of θ½V̇O2max that emerged as insufficient to elicit a similar cardiorespiratory and metabolic response in short HIIT. Therefore, a slight modification of the short HIIT design, i.e. shorter sprint with supramaximal exercise intensities, is recommended for training practice. Such alteration would also probably show a greater difference between endurance and sprint athletes as previously demonstrated by Ufland et al. (2013). Endurance athletes performed all the exercise interventions with a moderately (CE) to largely (both HIIT formats) higher mean V̇O2 than sprint athletes, corresponding to the higher performance achieved in GXT. Since the mean, however, of the individually prescribed running speed was moderately different and V̇O2max was largely different between the groups of endurance and sprint athletes, the V̇O2 values were also expressed per running velocity or as % V̇O2max which brought about trivial or at the most small between-group differences (Figure 2). The substantially lower mean acute cardiorespiratory response to the constant load exercise in this investigation, when compared to HIITs, is in contrast to Tschakert et al. (2015) and might be considered unexpected since the mean power for all the exercise interventions was matched in advance. This fact could have been partly caused by short breaks (approx. 20 s) in running during CE for regular capillary blood withdrawal which made the actual final mean power 3.4 % lower than in both HIITs. This shortcoming of the constant load design did not, however, induce a substantial difference. When these breaks in running were removed from the statistical analysis, the mean HR was higher by 0.9 and 1.2 bpm for endurance and sprint athletes, respectively. Similarly, mean V̇O2 only increased by 1.0 mL O2.kg-1.min-1 for both study groups. The running speed prescribed at 50 % of θ½V̇O2max for the constant load was in all probability too low for these highly trained athletes to elicit a sufficient cardiorespiratory response.
Acute metabolic responseThe capillary blood lactate concentration increased remarkably after the warm-up in both HIIT interventions, particularly with the most pronounced increase in the long-interval format. As presented in classical physiological literature (Saltin and Essén, 1971), this higher metabolic response during the long HIIT was expected. Differences between endurance and sprint athletes were apparent later during the HIITs with likely/very likely moderately higher lactate concentrations in sprint athletes. Unlike endurance athletes, there was a gradual increase in the lactate concentrations during both HIIT formats in sprint athletes which indicated a missing balance between lactate production and lactate consumption. Endurance athletes manifested a lactate steady state or even switched to lactate elimination indicated by a slight (but not substantial) lactate decrease in the long intervals. A moderate to large difference between endurance and sprint athletes was revealed regarding RER. Accepting the fact that energy production is provided to a substantial extent by anaerobic metabolic pathways when RER value exceeds the 1.0 level (Kenney et al., 2015), it is apparent (Figure 3) that in endurance athletes only the first interval considerably stimulated the anaerobic glycolytic system while the second to fourth interval was mostly aerobic also indicated by a decreased net lactate increase in blood. In contrast, the first three intervals in sprint athletes relied more on anaerobic lactate production. The higher aerobic capacity in endurance athletes definitely plays an important role and in all probability explains this fact (Hetlelid et al., 2015). However, it needs to be mentioned that RER might also be influenced by other factors, such as dietary fat intake, muscle glycogen content and circulating substrates (Venables, Achten and Jeukendrup, 2005). The progressive RER decrease and its peak values below the 1.0 level in later intervals, particularly in endurance athletes, raises the question as to the meaning of such a HIIT session. Coaches and athletes need to be cautious if the development of the glycolytic metabolic pathway is the main aim of such a training program. It has previously been demonstrated that an elevated H+ from a first high intensity interval may completely inhibit glycogen phosphorylase activity (glycogenolytic flux) and simultaneously maintain a high level of pyruvate dehydrogenase activity during subsequent high intensity intervals which means a shift towards a greater reliance on oxidative phosphorylation (Parolin et al., 1999). Contradictorily to the presented results, this explanation would mean that the sprint athletes manifesting higher lactate values will have reduced mean RER. However, the RER decrease is obviously more pronounced in the sprint athletes but remaining higher at the end due to the high initial values in the first exercise interval (Figure 3). It is worth mentioning that the metabolic response to short HIIT was close to the response to CE, which is in accordance with Wallner et al. (2014) and supports characterizing such short HIIT as mostly aerobically balanced exercise even if the cardiorespiratory and lactate responses oscillate intensively in the short HIIT compared to CE. Our results indicated, however, that this is only valid for endurance trained athletes since a steady state during short HIIT was not achieved amongst the majority of the sprint athletes. This finding does not signify that the short HIIT and constant load exercise can be interchangeable, since a higher performance improvement after HIIT has previously been observed (Helgerud et al., 2007).
Post-exercise HRVThe post-exercise cardiac autonomic regulation, assessed by HRV, is a compound measure influenced by a number of factors such as blood pressure regulation, baroreflex activity and primarily metaboreflex which drives sympathetic withdrawal and parasympathetic reactivation (Stanley et al., 2013; Buchheit, 2014). Since the anaerobic contribution appears to be of primary importance in determining the level of parasympathetic reactivation, post-exercise HRV might be viewed as a marker of exercise intensity (Buchheit et al., 2007) as well as cardiovascular homeostasis restoration (Stanley et al., 2013). The post-exercise ln rMSSD decrease was considerable in both HIIT formats. Since the metaboreflex is the most important post-exercise HRV determinant in high intensity exercise (Buchheit, 2014), the observed more extensive ln rMSSD decrease after long HIIT is predictable. The ln rMSSD values, however, were almost completely restored 1 h after the exercise. The differences between endurance and sprint athletes in response to the exercise interventions were not substantial (Figure 5A). This finding is in contrast to Stanley et al. (2013) or Seiler et al. (2007) who demonstrated a close association between the fitness level and the magnitude of the reduction of the post-exercise cardiac parasympathetic modulation as well as in the speed of restoration. Specifically, the higher the fitness level, the lower the post-exercise cardiac autonomic suppression and the shorter the time needed for recovery is suggested. This indicates that the difference in cardiorespiratory fitness between endurance and sprint type athletes needs to be much more pronounced to induce a meaningful difference in the post-exercise cardiac autonomic restoration (Cipryan, 2016a).
Interleukin-6 (IL-6) and leucocytesThe immediate post-exercise increases in IL-6 and leucocytes were evident following all exercise interventions. These responses were much greater in HIIT trials than that of the continuous exercise of the same duration and mean power (Figure 5B-C). In agreement with a recent study (Cullen et al., 2016), it is apparent that exercise intensity significantly influences the IL-6 response. The magnitude of the IL-6 increase reported in this study was, however, considerably smaller than those observed following exercise of a longer duration such as a marathon (Suzuki et al., 2003) indicating exercise duration as an additional crucial factor (Fischer, 2006). As regards the primary aim of this study, there were almost no substantial differences between endurance and sprint athletes in the post-exercise IL-6 and leucocytes concentration changes. The only exceptions were small to moderate differences in the PRE vs. 1h comparison for the short HIIT (both IL-6 and leucocytes) and PRE vs. POST for the long HIIT. Since the exercise induced inflammation might also be considered an important factor for eliciting a training adaptation (Pedersen and Febbraio, 2012; Nieman et al., 2015), the presented results demonstrated a similar response to these training stimulus between endurance and sprint trained athletes, at least from this point of view. The considerable inter-individual variation in IL-6, particularly in endurance athletes, needs to be pointed out.
Creatine kinase (CK) and myoglobinExercise-induced muscle damage occurs following unaccustomed and/or very vigorous exercise. Even if a ‘gold standard’ for exercise-induced muscle damage assessment has not been established yet in human research, various markers are usually applied. Apart from other factors (e.g. histological observation, changes in muscle force-generating capacity), increased levels of CK and myoglobin rank among those signs of exercise-induced muscle damage (Paulsen et al., 2012). Since its severity also depends on exercise intensity (Chen et al., 2007) evaluation of the CK and myoglobin changes in response to exercise might be helpful for the presented holistic approach of distinguishing the differences between endurance and sprint athletes. All the differences between the study groups in response to the exercise interventions were trivial for CK and small to at most moderate or not substantial for myoglobin. Neither the above-mentioned physiological variables nor the CK and myoglobin measures revealed any considerable differences between endurance and sprint type athletes that could be easily generalized.
Practical applications
This study has demonstrated that endurance athletes are able to perform both HIIT formats (short and long work intervals) with increased reliance on aerobic metabolic pathways although exercise intensity was identical in relative terms for all the participants. Despite this difference, the mean V̇O2 (expressed as a percentage of V̇O2max) and HR as well as markers of the cardiac autonomic regulation, systemic inflammation and muscle damage monitored during the early recovery phase did not demonstrate any differences between endurance and sprint trained individuals.
ACKNOWLEDGEMENTS |
This work was supported by Ostrava University under Grant SGS02/PdF2015. The authors would like to kindly thank B. Kawulokova, M. Krayzlova, P. Nemcik and S. Matyskova for assisting during data collection and Dr. Z. Svagera for the contribution with biochemical analysis. The study experiment complies with the current laws of the Czech Republic and Austria. All authors declare no conflict of interest. |
|
AUTHOR BIOGRAPHY |
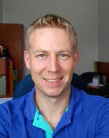 |
Lukas Cipryan |
Employment: Lecturer & Researcher, Human Motion Diagnostic Centre & Department of Human Movement Studies, Ostrava University, Czech Republic |
Degree: PhD |
Research interests: Exercise physiology, performance diagnostics and training, exercise prescription |
E-mail: Lukas.cipryan@osu.cz |
|
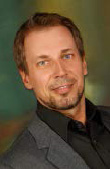 |
Gerhard Tschakert |
Employment: Ass.-Professor at the University of Graz Institute of Sports Science |
Degree: Dr. rer. nat. |
Research interests: Exercise physiology, exercise prescription, exercise testing, performance diagnostics, and training, training therapy |
E-mail: gerhard.tschakert@uni-graz.at |
|
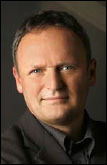 |
Peter Hofmann |
Employment: Professor at the University of Graz |
Degree: Dr. rer. nat. |
Research interests: Exercise testing and performance diagnostics, exercise prescription and training, training therapy |
E-mail: peter.hofmann@uni-graz.at |
|
|
|
REFERENCES |
 Åstrand I., Åstrand P.-O., Christensen E.H., Hedman R. (1960) Intermittent Muscular Work. Acta Physiologica Scandinavica 48, 448-453.
|
 Batterham A.M., Hopkins W.G. (2006) Making Meaningful Inferences About Magnitudes. International Journal of Sports Physiology and Performance 1, 50-57.
|
 Berger N.J.A., Jones A.M. (2007) Pulmonary O Uptake On-Kinetics in Sprint- and Endurance-Trained Athletes. Applied Physiology, Nutrition, and Metabolism 32, 383-393.
|
 Bosco C., Luhtanen P., Komi P.V. (1983) A Simple Method for Measurement of Mechanical Power in Jumping. European Journal of Applied Physiology and Occupational Physiology 50, 273-282.
|
 Buchheit M (2014) Monitoring Training Status with HR Measures: Do All Roads Lead to Rome?. Frontiers in Physiology 5, 73-.
|
 Buchheit M., Laursen P.B. (2013) High-Itensity Interval Training, Solutions to the Programming Puzzle: Part II: Anaerobic Energy, Neuromuscular Load and Practical Applications. Sports Medicine 43, 927-954.
|
 Buchheit M., Laursen P.B., Ahmaidi S. (2007) Parasympathetic Reactivation after Repeated Sprint Exercise. AJP: Heart and Circulatory Physiology 293, H133-H141.
|
 Chen T., Nosaka K., Sacco P. (2007) Intensity of Eccentric Exercise, Shift of Optimum Angle, and the Magnitude of Repeated-Bout Effect. Journal of Applied Physiology 102, 992-999.
|
 Cipryan L (2016a) The Effect of Fitness Level on Cardiac Autonomic Regulation, IL-6, Total Antioxidant Capacity, and Muscle Damage Responses to a Single Bout of High-Intensity Interval Training. Journal of Sport and Health Science , -.
|
 Cipryan L (2016b) Within-Session Stability of Short-Term Heart Rate Variability Measurement. Journal of Human Kinetics 50, 85-92.
|
 Cipryan L., Laursen P.B., Plews D.J. (2016) Cardiac Autonomic Response Following High-Intensity Running Work-to-Rest Interval Manipulation. European Journal of Sport Science 16, 808-817.
|
 Cullen T., Thomas A.W., Webb R., Hughes M.G. (2016) Interleukin-6 and Associated Cytokine Responses to an Acute Bout of High-Intensity Interval Exercise: The Effect of Exercise Intensity and Volume. Applied Physiology, Nutrition, and Metabolism 41, 803-808.
|
 Dubouchaud H., Butterfield G.E., Wolfel E.E., Bergman B.C., Brooks G. (2000) Endurance Training, Expression, and Physiology of LDH, MCT1, and MCT4 in Human Skeletal Muscle. American journal of physiology. Endocrinology and metabolism 278, 571-579.
|
 Fischer C.P. (2006) Interleukin-6 in Acute Exercise and Training: What Is the Biological Relevance?. Exercise Immunology Review 12, 6-33.
|
 Haff G.G., Dumke C. (2012) Laboratory Manual for Exercise Physiology. Champaign. Human Kinetics.
|
 Hawley J.A. (2002) Adaptations of Skeletal Muscle to Prolonged, Intense Endurance Training. Clinical and Experimental Pharmacology and Physiology 29, 218-222.
|
 Helgerud J., Hoydal K., Wang E., Karlsen T., Berg P., Bjerkaas M., Simonsen T., Helgesen C., Hjorth N., Bach R., Hoff J. (2007) Aerobic High-Intensity Intervals Improve VO More than Moderate Training. Medicine & Science in Sports & Exercise 39, 665-671.
|
 Hetlelid K.J., Plews D.J., Herold E., Laursen P.B., Seiler S. (2015) Rethinking the Role of Fat Oxidation: Substrate Utilisation during High-Intensity Interval Training in Well-Trained and Recreationally Trained Runners. BMJ Open Sport & Exercise Medicine 1, e000047-.
|
 Hofmann P., Tschakert G. (2011) Special Needs to Prescribe Exercise Intensity for Scientific Studies. Cardiology Research and Practice 2011, 1-10.
|
 Hopkins W.G. (2006) Spreadsheets for Analysis of Controlled Trials, with Adjustment for a Subject Characteristic. Sportscience 10, 46-50.
|
 Hopkins W.G., Batterham A.M. (2016) Error Rates, Decisive Outcomes and Publication Bias with Several Inferential Methods. Sports Medicine 46, 1563-1573.
|
 Hopkins W.G., Marshall S.W., Batterham A.M., Hanin J. (2009) Progressive Statistics for Studies in Sports Medicine and Exercise Science. Medicine & Science in Sports & Exercise 41, 3-13.
|
 Kenney W.L., Wilmore J.H., Costill D. (2015) Physiology of Sport and Exercise. Champaign. Human Kinetics.
|
 Kohn T.A., Essén-Gustavsson B., Myburgh K.H. (2011) Specific Muscle Adaptations in Type II Fibers after High-Intensity Interval Training of Well-Trained Runners. Scandinavian Journal of Medicine & Science in Sports 21, 765-772.
|
 Malik M., Bigger J., Camm A., Kleiger R. (1996) Heart Rate Variability. Standards of Measurement, Physiological Interpretation, and Clinical Use. Task Force of the European Society Of Cardiology and the North American Society of Pacing and Electrophysiology. European Heart Journal 17, 354-381.
|
 Milanović Z., Sporiš G., Weston M. (2015) Effectiveness of High-Intensity Interval Training (HIT) and Continuous Endurance Training for VO Improvements: A Systematic Review and Meta-Analysis of Controlled Trials. Sports Medicine 45, 1469-1481.
|
 Nieman D.C., Zwetsloot K.A., Meaney M.P., Lomiwes D.D., Hurst S.M., Hurst R.D. (2015) Post-Exercise Skeletal Muscle Glycogen Related to Plasma Cytokines and Muscle IL-6 Protein Content, but not Muscle Cytokine mRNA Expression. Frontiers in Nutrition 2, 27-.
|
 Nuzzo R (2014) Statistical Errors. Nature 506, 150-152.
|
 Parolin M.L., Chesley A, Matsos M.P., Spriet L.L., Jones N.L., Heigenhauser G.J. (1999) Regulation of Skeletal Muscle Glycogen Phosphorylase and PDH During Maximal Intermittent Exercise. The American Journal of Physiology 277, E890-E900.
|
 Paulsen G., Mikkelsen U.R., Raastad T., Peake J.M. (2012) Leucocytes, Cytokines and Satellite Cells: What Role Do They Play in Muscle Damage and Regeneration Following Eccentric Exercise?. Exercise Immunology Review 18, 42-97.
|
 Pedersen B.K., Febbraio M.A. (2012) Muscles, Exercise and Obesity: Skeletal Muscle as a Secretory Organ. Nature Reviews Endocrinology 8, 457-465.
|
 Penttila J., Helminen A., Jartti T., Kuusela T. (2001) Time Domain, Geometrical and Frequency Domain Analysis of Cardiac Vagal Outflow: Effects of Various Respiratory Patterns. Clinical Physiology 1, 365-376.
|
 Plews D.J., Laursen P.B., Stanley J., Kilding A.E., Buchheit M. (2013) Training Adaptation and Heart Rate Variability in Elite Endurance Athletes: Opening the Door to Effective Monitoring. Sports Medicine 43, 773-781.
|
 Saltin B., Essén B., Pernow B., Saltin B. (1971) Muscle Metabolism During Exercise. Muscle Glycogen, Lactate, ATP, And CP in Intermittent Exercise. US. Springer.
|
 Seiler S., Haugen O., Kuffel E. (2007) Autonomic Recovery after Exercise in Trained Athletes. Medicine & Science in Sports & Exercise 39, 1366-1373.
|
 Spencer M., Bishop D., Dawson B., Goodman C. (2005) Physiological and Metabolic Responses of Repeated-Sprint Activities. Sports Medicine 35, 1025-1044.
|
 Stanley J., Peake J.M., Buchheit M. (2013) Cardiac Parasympathetic Reactivation Following Exercise: Implications for Training Prescription. Sports Medicine 43, 1259-1277.
|
 Suzuki K., Nakaji S., Yamada M., Liu Q., Kurakake S., Okamura N., Kumae T., Umeda T., Sugawara K. (2003) Impact of a Competitive Marathon Race on Systemic Cytokine and Neutrophil Responses. Medicine & Science in Sports & Exercise 35, 348-355.
|
 Thomas C., Sirvent P., Perrey S., Raynaud E., Mercier J. (2004) Relationships between Maximal Muscle Oxidative Capacity and Blood Lactate Removal after Supramaximal Exercise and Fatigue Indexes in Humans. Journal of Applied Physiology 97, 2132-2138.
|
 Tschakert G., Hofmann P. (2013) High-Intensity Intermittent Exercise: Methodological and Physiological Aspects. International Journal of Sports Physiology and Performance 8, 600-610.
|
 Tschakert G., Kroepfl J., Hofmann P., Mueller A., Moser O., Groeschl W. (2015) How to Regulate the Acute Physiological Response to “Aerobic” High-Intensity Interval Exercise. Journal of Sports Science and Medicine 14, 29-36.
|
 Ufland P., Ahmaidi S., Buchheit M. (2013) Repeated-Sprint Performance, Locomotor Profile and Muscle Oxygen Uptake Recovery: Effect of Training Background. International Journal of Sports Medicine 34, 924-930.
|
 Venables M.C., Achten J., Jeukendrup A.E. (2005) Determinants of Fat Oxidation during Exercise in Healthy Men and Women: a Cross-Sectional Study. Journal of Applied Physiology 98, 160-167.
|
 Wallner D., Simi H., Tschakert G., Hofmann P. (2014) Acute Physiological Response to Aerobic Short-Interval Training in Trained Runners. International Journal of Sports Physiology and Performance 9, 661-666.
|
 Wasserstein R.L., Lazar N.A. (2016) The ASA’s Statement on p -Values: Context, Process, and Purpose. The American Statistician 70, 129-133.
|
 Zierath J.R., Wallberg-Henriksson H. (2015) Looking Ahead Perspective: Where Will the Future of Exercise Biology Take Us?. Cell Metabolism 22, 25-30.
|
|
|
|
|
|
|