|
|
|
ABSTRACT |
We investigated the acute effects of dynamic stretching on mechanical properties of plantar flexor muscles and tested the hypothesis that it would result from an interaction between muscle-tendon stretching and muscle warm-up. To test the stretching effect, dynamic stretching (DS) was compared to static stretching (SS). To test the warm-up effect, DS was compared to submaximal isometric muscle activity (SIMA) with similar contraction intensity. A control condition served as reference. These four conditioning activities were time matched (2×20s) and tested on separate days on 13 volunteers. Electrical neurostimulation was applied to investigate muscle mechanical properties (peak doublet torque (PDT) and rate of torque development (RTD)), before assessing maximal voluntary isometric torque (MVIT). Passive torque and muscle fascicles length, were measured respectively with a dynamometer and ultrasonography during a maximal passive dorsiflexion. These parameters were recorded before and after each conditioning activities. MVIT, PDT, RTD and fascicles length remained unaffected, while passive torque was significantly reduced (-11.6 ± 14.8%) after DS. SS significantly decreased MVIT (-7.7 ± 3.8%) and enhanced fascicles length (45.3 ± 49.2%), whereas SIMA increased PDT (5.8 ± 5.2%) and RTD (7.4 ± 8.3%) without affecting passive torque or fascicles length. These results may suggest that, during dynamic stretching, muscle-tendon stretching effects would partly counteract muscle warm-up effects. |
Key words:
Pre-exercise intervention, muscle stretching, submaximal contractions, passive resistive torque, fascicles extensibility, contractile properties
|
Key
Points
- Dynamic stretching did not affect maximal voluntary and evoked torque production, but decreased passive resistive torque.
- During dynamic stretching, MTU stretching effects would partly counteract muscle warm-up effects.
- Associated to stronger contractions, dynamic stretching could be a part of warm-up procedure, to optimize the subsequent muscle performance.
|
Static stretching is usually involved in pre-exercise routines in sport and rehabilitation environments (Behm et al., 2016), in order to enhance range of motion (ROM) around a joint (Young and Behm, 2002). However, a large body of evidence reported that it may acutely impair the subsequent muscular performance, reducing maximal voluntary strength, muscle power or balance (Behm and Chaouachi, 2011). Theses impairments could originate from neural (reduced central drive (Budini et al., 2017; Trajano et al., 2017)) and peripheral (altered intrinsic muscular properties and musculotendinous unit (MTU) stiffness (Opplert et al., 2016)) mechanisms. Alternatively, dynamic stretching, consisting of muscle contractions to move the joint through a full active ROM, is also incorporated as pre-exercise interventions (Opplert and Babault, 2018). In addition, recent studies revealed that dynamic stretching may provide an acute increase in muscular contractility (Yamaguchi et al., 2007), sprints, jumps or even balance (Opplert and Babault, 2018). Therefore, such a modality appears to be more favorable than prolonged static stretching to prepare the musculotendinous system for subsequent exercises. These two pre-exercise activities might be expected to impact differently the MTU stiffness and thus the state of the muscle-tendon system for subsequent exercises. Indeed, it is known that mechanical effects of stretching could be mediated by a number of stretch characteristics, including amplitude and muscular activation (Taylor et al., 1990; Knudson, 2007), which obviously differ between static and dynamic stretching. For instance, static stretching implies a greater load of MTU than dynamic stretching. It results from a greater ROM and the stress relaxation which occurs when the muscle is kept stretched in a fixed position (Magnusson et al. 1996b). Thus, static stretching would likely involve larger changes in stiffness of different tissues, especially muscle fascicles (Mizuno et al., 2013). Moreover, because muscles are contracting actively and rhythmically, dynamic stretching may increase muscle fibers temperature (Fletcher, 2010) and thus lower viscosity (Mutungi and Ranatunga, 1998; Bishop, 2003a). Indeed, it has been shown that repetitive muscle contractions, such as those produced during warm-up procedures, also influenced MTU mechanical properties (Bishop, 2003b, a). In addition, repetitive movements and contractions may also induce improved muscle contractility, mainly attributable to temperature- and/or potentiation-related mechanisms (Bishop, 2003a; Turki et al., 2011). Consequently, the influence of dynamic stretching on viscoelastic and contractile properties seems to result from both the stretching of muscle-tendon structures and muscle warm-up. However, how these two mechanisms interact and specifically impact the mechanical properties remains unexplored. We aimed to test the hypothesis that dynamic stretching would result from an interaction between MTU stretching and muscle warm-up. Therefore, the effects of MTU stretching have been studied through the comparison between static and dynamic stretching, while the muscle warm-up effects were investigated comparing dynamic stretching to submaximal isometric muscle activity (of a similar intensity). To test this hypothesis, this study explored mechanical properties of plantar flexor muscles through contractile properties, passive resistive torque and muscle fascicles extensibility.
Thirteen healthy recreationally active men (mean ± standard deviation: age 24.9 ± 2.5 years; height 1.81 ± 0.05 m; body mass 82.3 ± 11.6 kg; 6.5 ± 2.5 h of physical activity per week, such as soccer, rugby or handball) without recent injury or illness were recruited for this study (see the statistical analysis section for the statistical power values). They were all volunteers and gave their written consent to participate in the experiment after being informed about the investigation. The study conformed to the standards set by the World Medical Association Declaration of Helsinki “Ethical Principles for Medical Research Involving Human Subjects” (2008) and approval was obtained from the local committee on human research (EAST-1, number: 2017-72).
Experimental procedureThis study used a randomized and cross-over design with control condition to assess the effects of three conditioning interventions on mechanical properties of plantar flexor muscles. Participants attended the laboratory on five separate occasions. The first session served as familiarization in order to practice the different conditioning activities and the testing procedures. Four 2 × 20-s experimental sessions including (i) static stretching (SS), (ii) dynamic stretching (DS), (iii) submaximal isometric muscle activity (SIMA), and (iv) control (CON) were completed in a random order with at least 48 h between. Testing procedures were conducted immediately before (pre-conditioning tests) and 10 s after (post-conditioning tests) the conditioning activities to quantify changes in evoked contractile properties, maximal voluntary isometric torque, passive resistive torque and muscle fascicles extensibility (Figure 1). Limb dominance was ignored and all experimental procedures were conducted on the right plantar flexor muscles. Conditioning activities and testing procedures were performed on an isokinetic dynamometer (Biodex System 4, BIODEX Corporation, Shirley NY, USA). To avoid gravitational influences, subjects laid on their left side (Figure 2). The contralateral leg was flexed (about 90°) for comfort and the right leg fully extended (0°) to ensure that the plantar flexor muscles were placed under significant stretch (Cresswell et al., 1995). To maintain this position, the right knee was positioned and secured on a dynamometer support. To minimize heel displacements, the foot was positioned and fastened inside a shoe (adapted to participant’s size and fixed by the sole to the dynamometer footplate), and firmly attached to the footplate of the dynamometer with straps. The lateral malleolus was aligned to the center of rotation of the dynamometer. From here, subjects were in a standardized position for ~20-min. The passive maximal range of motion was first determined by the experimenter, stretching slowly the plantar flexor muscles from a maximal plantar flexion until the point of maximal tolerated discomfort (ROMend), and returned immediately to a neutral position (0°). Then and for all four experimental sessions, subjects were asked to perform 5 repetitions of dynamic stretching (see below for DS procedure). These repetitions aimed to evaluate muscle electromyographic (EMG) activity during DS for reproduction during SIMA conditioning activity (see below for SIMA procedure). Thereafter, while the subjects remained relaxed in the neutral position, the ultrasound probe was positioned and the M-wave recruitment curve was established. Then, a short warm-up of ten incremental submaximal voluntary contractions was performed and followed by a rest period of 5 min.
Conditioning activitiesAll conditions were time matched for direct comparison. The static stretching (SS) intervention included two sets of static stretching. The subjects’ ankle was passively rotated using the isokinetic dynamometer with a standardized 25° of ROM until individual ROMend, in order to match the lengthening duration of the static stretches. A slow angular velocity (5 °·s-1) was set in order to avoid the myotatic reflex (Kay and Blazevich 2010) to obtain a good resolution of the fascicles via ultrasonography. Each set of static stretching took 20 s, including 5 s of lengthening, a 15-s hold before the ankle was released immediately to the start position at 60 °·s-1 in readiness for the next stretch (20-s between-stretch rest). The subjects were instructed to relax during the stretching, and not to offer any resistance to the dynamometer, which was controlled with EMG activity of SOL and GL muscles. For the dynamic stretching (DS) condition, the subjects were instructed to pull their right foot towards maximal dorsiflexion as fast as possible against the resistance provided by the mechanical inertia of the isokinetic dynamometer, and to extend their foot back towards maximal plantar flexion at a self-regulated pace that permitted a dorsi-plantar flexions cycle frequency of 1 Hz set by a metronome. Two 20 s sets, including 20 cycles (20-s between-set rest), were performed through a full active ROM, for a total stretch procedure of 60 s. The submaximal isometric muscle activity (SIMA) condition was performed in a neutral position, to avoid any MTU stretching. It consisted of replicating the EMG activity of plantar flexors occurring during the plantar flexions of dynamic stretching, using the mean root mean squared (RMS) value displayed on-screen as a target line. During 20 s, participants had to perform short plantar flexors voluntary isometric contractions for a frequency of 1Hz during two sets of 20 s (20-s between-set rest). During the control condition (CON), the subjects were instructed to relax with the ankle held in the neutral position for a duration corresponding to the stretching protocol (60 s). Electromyographic activity (EMG values) was registered from the soleus (SOL) and the gastrocnemius lateralis (GL) to control contraction intensities during conditioning activities. The belly of GM muscle being mostly covered by the ultrasound probe, the EMG signals were not collected for this muscle. The skin under the electrodes was shaved and dry cleaned with alcohol to obtain low impedance (<5 kθ©). EMG signals were recorded by two pairs of silver-chloride electrodes (10-mm diameter) with an interelectrode distance of 2 cm. Electrodes for SOL were applied 2 cm below the intersection of the gastrocnemii over the Achilles tendon. For GL, electrodes were placed over the mid-belly of the muscle. The reference electrode was pasted over the contralateral patella. EMG signals were amplified with a bandwidth frequency ranging from 10 Hz to 5 kHz (gain = 500). EMG signals (and also torque traces) were recorded with a Biopac MP150 system (Biopac System, Santa Barbara, CA) at a sampling rate of 2 kHz, and stored for analysis with the AcqKnowledge software (AcqKnowledge 4.2 for MP systems, Biopac System, Santa Barbara, CA). EMG activity during plantar flexion movements (for ~350 ms) was quantified using RMS values.
Testing procedureThe testing procedure (total duration = ~40 s) was conducted immediately before and 10 s after the intervention. Two doublets (two single electrical impulses delivered successively at 100 Hz) were applied at rest with 10-s interval in between. Ten seconds after these doublets, a single 5-s maximal voluntary isometric contraction (MVIC) was performed. The ankle was set in the neutral position for these assessments. To investigate passive resistive torque and muscle fascicles extensibility, the subjects’ ankle was passively rotated from the neutral position until the maximal dorsiflexion, previously determined, at 5 °·s-1; a slow angular velocity was set in order to avoid the myotatic reflex (Blazevich et al. 2014), which ensured that torque data were observed under passive conditions. Without holding the stretched position, the ankle returned immediately in a neutral position. Tests were performed in an inter-individual randomized order, which remained constant during each session for a same subject. Electrical neurostimulation was applied to investigate plantar flexors contractile properties. The posterior tibial nerve was stimulated with single rectangular pulses (1 ms) using a Digitimer stimulator (DS7A, Hertfordshire, UK) with the ankle in the neutral position. The cathode (10 mm diameter) was placed in the popliteal fossa and the anode (5 × 10 cm) to the patella on the anterior surface of the knee. The optimal stimulation site was determined by eliciting, with a hand-held stimulation probe, the greatest amplitude of SOL M-wave for a given intensity. Then, the stimulation electrode was pasted to the site. The stimulation intensity was increased by 5 mA increments from 0 mA to the maximal SOL M-wave (Mmax), in order to build the recruitment curve and determine the intensity used for contractile properties assessments. For the recruitment curve, two stimulations were delivered at each intensity with a 10-s interval between stimuli. During the testing procedure, stimulations were applied at a supramaximal stimulus intensity (120% Mmax intensity) (Ryan et al. 2008a). Fascicles extensibility of gastrocnemius medialis (GM) muscle was investigated via measurements of fascicles length using a B-mode ultrasound video imaging (AU5; Esaote Biomedica, Florence, Italy). A 7.5 MHz linear-array probe was oriented along the longitudinal axis of the MTU and held at 50% of GM muscle length in the mid-sagittal line. The probe was then firmly attached over the skin in this position with a custom-made apparatus, which permitted a constant pressure between the probe and the dermal surface. An echo-sensitive wire serving as a marker was placed under the probe and pasted over the skin to check for a constant probe position.
Data analysisFrom the mechanical responses elicited by doublets were calculated the peak doublet torque (PDT) and the maximal rate of torque development (RTD, maximal value measured from the torque first derivate). These values were determined as the highest value of the two doublets. Additionally, maximal voluntary isometric torque (MVIT) was recorded from the MVIC. Dynamometer and ultrasound data were synchronized using a custom-made trigger to ensure that passive torque and fascicles length were measured every five degrees during the final 15° until ROMend (Herda et al. 2011, 2012). Accordingly, variation of passive torque was quantified as a difference between values obtained from 15 to 10° (noted 15-10°), from 10 to 5° (noted 10-5°) and from 5° to ROMend (noted 5°-ROMend). Finally, the difference between values obtained from 15° to ROMend (noted 5°-ROMend) was also calculated. Similarly, variation of fascicles length was quantified, using an open source digital measurement software (Image J, NIH, USA). When fascicles extended off the acquired ultrasound image, the length of the missing portion of the fascicle was estimated by extrapolating linearly both the fascicular path, visible in the image, and the aponeurosis. Length variations from three fascicles per passive stretch were measured and then averaged.
Statistical analysisStatistical analyses were performed with Statistica (StatSoft, version 7.1, Tulsa, OK). The normality of all data was tested and confirmed by the Shapiro-Wilk test. A two-way [conditioning activity (SS vs. DS vs. SIMA vs. CON) × time (pre- vs. post-intervention tests)] analysis of variance (ANOVA) with repeated measures was used on absolute values. From main effects or interactions of the ANOVA were calculated partial eta squared (ηp2) with values of 0.01, 0.06 and above 0.14 represented small, medium and large differences, respectively (Cohen J 1988). When significant main effects or interactions were present, Student Newman-Keuls (SNK) post-hoc tests were conducted. A one-way ANOVA with repeated measures was used to compare relative changes (percentage of pre-intervention tests) between conditioning activities. When significant conditioning activity effects were present, SNK post-hoc tests were subsequently conducted. Statistical significance was accepted at P<0.05. Exact P values were specified, except for values <0.001. Statistical power for all comparisons ranged from 0.23 to 0.99. Subsequently, Cohen’s qualitative descriptors of standardized effects were used for pair-wise comparison, such that effect sizes (ES) <0.4, 0.41–0.7 and >0.7 represented small, moderate and large magnitudes of change, respectively (Cohen J 1988). Absolute values are expressed as mean ± standard deviations (SD) and relative changes as percentage of pre-intervention tests ± standard deviations (SD).
Statistical analyses revealed a significant main conditioning activity effect for the averaged range of dorsiflexion reached during stretching (p <0.001, ηp2 = 0.79), which was significantly greater during SS (39.8 ± 8.7°) in comparison with DS (30.9 ± 8.8°) (p <0.001, ES = 1.02). For PDT and RTD, the two-way ANOVA indicated a conditioning activity and time interaction (p <0.001, ηp2 = 0.36 and p = 0.023, ηp2 = 0.23 for PDT and RTD, respectively). Post-hoc analyses only revealed that PDT and RTD significantly increased after SIMA as compared to pre-intervention (p = 0.001, ES = 0.31 and p = 0.002, ES = 0.41; respectively) (Table 1). When considering relative changes, the one-way ANOVA revealed a significant main conditioning activity effect (p <0.001, ηp2 = 0.38 and p = 0.012, ηp2 = 0.26 for PDT and RTD, respectively), showing an increase in PDT and RTD after SIMA as compared to SS (p <0.001, ES = 1.85 and p = 0.01, ES = 0.99; respectively), DS (p <0.001, ES = 1.31 and p = 0.007, ES = 0.93; respectively) and CON (p = 0.001, ES = 1.67 and p = 0.003, ES = 1.27; respectively). The two-way ANOVA for MVIT indicated a conditioning activity and time interaction (p = 0.003, ηp2 = 0.32). MVIT significantly decreased after SS as compared to pre-intervention tests (p <0.001, ES = 0.43). Relative changes revealed a significant main conditioning activity effect (p = 0.002, ηp2 = 0.33); MVIT was significantly decreased after SS relative to DS (p = 0.005, ES = 1.26) and SIMA (p = 0.003, ES = 1.23) and CON (p <0.001, ES = 1.55). A significant main time effect was reported for passive torque variation (15-10°) (p <0.001, ηp2 = 0.65), (10-5°) (p = 0.002, ηp2 = 0.58), (5°-ROMend) (p = 0.002, ηp2 = 0.55) and (15°-ROMend) (p <0.001, η2 = 0.8) with a reduction from pre- to post-intervention (p = 0.002, ES = 0.18; p <0.001, ES = 0.3; p <0.001, ES = 0.21 and p = 0.002, ES=0.16; respectively). In addition, statistical analyses revealed a conditioning activity and time interaction (p = 0.01, ηp2 = 0.26) for passive torque variation during the last degrees (5°-ROMend), which was significantly decreased after DS in comparison with pre-intervention (p <0.001, ES = 0.47). When considering relative changes, the one-way ANOVA indicated a significant main conditioning activity effect (p = 0.014, ηp2 = 0.25); passive torque variation (5°-ROMend) was reduced after DS as compared to SS (p = 0.011, ES = 0.92) and CON (p = 0.003, ES = 1.04) (Table 2). Passive torque variation recorded on other parts of ROM (15-10°, 10-5° and 15°-ROMend) remained unaffected after DS. The two-way ANOVA for fascicle length variations recorded during the last degrees (5°-ROMend) and total ROM (15°-ROMend) revealed a conditioning activity and time interaction (p <0.001, ηp2 = 0.39 and p <0.001, ηp2 = 0.36, respectively). Post-hoc analyses only exposed that fascicle length variations (5°-ROMend) and (15°-ROMend) significantly increased after SS as compared to pre-intervention tests (p <0.001, ES=1.05 and p <0.001, ES = 1.01; respectively) (Table 2). Relative changes indicated a main conditioning activity effect (p <0.001, ηp2 = 0.4 and p <0.001, ηp2 = 0.42, respectively); fascicle length variations (5°-ROMend) and (15°-ROMend) significantly increased after SS in comparison with DS (p <0.001, ES = 1.25 and p <0.001, ES = 1.17; respectively), SIMA (p <0.001, ES = 1.04 and p <0.001, E S=0.95; respectively) and CON (p <0.001, ES = 1.28 and p <0.001, ES = 1.45; respectively). Fascicles length variation measured on partial ROM (15-10°) and (10-5°) remained unaltered after SS.
The present study aimed to test the hypothesis that dynamic stretching would result from an interaction between MTU stretching and muscle warm-up. The results provided potential support for this assumption, because dynamic stretching did not affect maximal voluntary and evoked torque production, as well as fascicles extensibility, while decreased passive resistive torque. Conversely, static stretching significantly reduced MVIT and enhanced fascicles extensibility, whereas submaximal isometric muscle activity increased evoked contractile properties (PDT and RTD) without affecting viscoelastic properties.
Stretching effectsOur data revealed an alteration of MVIT after static stretching, which is congruent with a part of the literature that has found decreases in maximal voluntary torque after similar static stretching durations (Behm et al., 2004; Power et al., 2004). They could originate from various neural (Budini et al., 2017; Trajano et al., 2017) and peripheral mechanisms such as altered intrinsic muscular properties and viscoelastic properties (Mizuno et al., 2013; Opplert et al., 2016). However, our results did not reveal any influence of static stretching on PDT and RTD. Previous studies (Ryan et al., 2008a; Cannavan et al., 2012) have even reported similar results and suggested that such a stretch duration would not be sufficient to induce cellular damage of a magnitude large enough to impair evoked torque. In contrast, our findings revealed that dynamic stretching had no detectible influence on both maximal evoked (PDT, RTD) and voluntary (MVIT) torque. This is congruent with previous studies, which have demonstrated no adverse effects on force with dynamic stretching (Herda et al., 2008; Jaggers et al., 2008; Costa et al., 2014). Consistent with the literature, dynamic stretching appears to be less detrimental to the subsequent muscular performance than static stretching (Opplert and Babault, 2018). Further, passive torque variation remained statistically unaltered after static stretching, which is in disagreement with the most part of the literature (Yeh et al., 2005; Ryan et al., 2008b; Morse et al., 2008; Herda et al., 2014). However, these studies used constant-torque stretches while the present one applied constant-angle stretches. Constant-torque protocols have been demonstrated to induce greater changes in passive resistive torque (Herda et al. 2014). It would be mainly due to a muscle creep occurring during the stretch, which may place more tension on the MTU than a constant-angle stretching protocol (Gajdosik, 2001; Herda et al., 2014). Inversely, a constant angle static stretching may induce a stress relaxation response, likely reducing the tension on the MTU (Gajdosik 2001; Herda et al. 2014). Unlike static stretching, passive torque variation was significantly decreased after dynamic stretching. Similarly, McNair et al. (McNair et al., 2000) have compared viscoelastic responses to static and cyclic passive stretching and only reported a decline in MTU stiffness during cyclic passive stretching. It suggests that cycling stretching may be more effective to reduce passive resistive torque than static stretching. Authors proposed that a reorganization of the muscle-tendon tissue, and especially a redistribution of the more mobile constituents of collagen fibers, such as the polysaccharides and water, could occur more rapidly during cycling stretching (McNair et al., 2000; Kubo et al., 2001; Nordez et al., 2009). Ours results also revealed that fascicles length variation was increased after static stretching, which is in agreement with some previous studies (Morse et al., 2008; Bouvier et al., 2017), while it remained unaltered after dynamic stretching. As previously discussed, the magnitude of change in viscoelastic properties is load-dependent (Gajdosik 2001). For instance, some studies (Nordez et al., 2006; Herda et al., 2012) reported that the magnitude in passive stiffness decrease was larger at the end of the range of motion. Given that, it is understandable that changes in fascicles extensibility would be lower after dynamic stretching, which involves smaller amplitude than static stretching. To explain the current results, the viscoelastic stress relaxation occurring during static stretching appears to be a strong candidate for the increase in fascicles length variation (Magnusson et al., 1996a; 1997; Gajdosik, 2001). To summarize, static and dynamic stretching differentially modulated the viscoelastic properties. While cyclic motion probably explains the reduced passive resistive torque after dynamic stretching, stress relaxation that occurred during static stretching more likely affected muscle fascicles extensibility.
Warm-up effectsRecent studies revealed that dynamic stretching may provide an acute increase in subsequent muscular performance (Opplert and Babault, 2018), which could mainly be attributable to temperature- and/or potentiation-related mechanisms (Bishop, 2003a; Turki et al., 2011). To investigate the muscle warm-up effects, similar intensity contractions in unstretched position were performed. As presented before, dynamic stretching had no detectible influence on maximal evoked and voluntary torque, while submaximal isometric muscle activity enhanced PDT and RTD. Because post-activation potentiation would be more likely associated with high force activities (Maloney et al., 2014), temperature-related mechanisms seem to be more likely to explain these results. Indeed, heavier loadings would shorten the time to peak torque and increase the rate of torque development, increasing muscular force, power and speed in subsequent performance, relative to lighter loadings (Sale, 2002; Tillin and Bishop, 2009). Therefore, voluntary and repetitive light contractions in unstretched position appeared to be sufficient enough to improve slight but significant muscle contractile properties, probably by inducing a muscle warm-up process, increasing muscular and core temperature (Fletcher and Monte-Colombo, 2010). Furthermore, MVIT remained also unaffected following submaximal isometric muscle activity, underpinning that such an intensity of contractions was likely not sufficient to increase maximal voluntary torque. Lastly, due to the lack of change in PDT and RTD, dynamic stretching appears to be less efficient for the subsequent muscular performance than isometric muscle activity of a similar intensity. It could have been expected that repetitive muscle contractions may affect MTU stiffness through temperature-related mechanisms; by increasing muscle fibers temperature and thus lowering viscous resistance (Buchthal et al., 1944; Mutungi and Ranatunga, 1998; Bishop, 2003a), increasing muscle extensibility and reducing passive muscle stiffness (Mutungi and Ranatunga, 1998; Herda et al., 2012). Nevertheless, the present results demonstrate that submaximal isometric muscle activity is unlikely to affect passive resistive torque and/or fascicles extensibility, highlighted by the lack of change in passive torque and fascicles length variations. It could be related to the methodology used to evaluate passive torque variations, especially to the velocity of the dorsiflexion. According to Noonan et al. (Noonan et al., 1993), a lower lengthening velocity may attenuate the change in muscle stiffness induced by the enhanced temperature, compared to a greater velocity. This is important because the low velocity used in our study may explain the lack of change in viscoelastic properties after SIMA.
The present data demonstrated no effect of dynamic stretching on maximal voluntary and evoked torque production, in contrast to static stretching and submaximal isometric muscle activity, which respectively reduced the maximal voluntary torque and enhanced the maximal evoked torque. Thus, dynamic stretching appears to be less detrimental than static stretching, probably due to the distinct stretching characteristics (static vs. cycling stretching, passive vs. active movements), but less beneficial than submaximal isometric muscle activity, likely owing to the stretch-induced effects. These results provide potential support for the hypothesis that, during dynamic stretching, MTU stretching effects would partly counteract muscle warm-up effects. From this basis, we can suggest to term dynamic muscle activity instead of dynamic stretching. Finally, from a practical point of view, dynamic stretching could be a part of warm-up procedure, but should be associated to stronger contractions to optimize the improvement in muscle strength capacities.
ACKNOWLEDGEMENTS |
The authors have no conflicts of interest to declare. The results of the study are presented clearly, honestly, and without inappropriate data manipulation. The experiments comply with the current laws of the country in which they were performed. |
|
AUTHOR BIOGRAPHY |
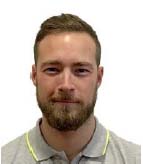 |
Jules Opplert |
Employment: Ph.D student in Sport Sciences, INSERM UMR1093-CAPS |
Degree: MSc |
Research interests: Stretching exercise, neuromuscular system |
E-mail: opplert.jules@gmail.com |
|
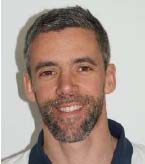 |
Nicolas Babault |
Employment: INSERM UMR1093-CAPS, Professor at the Sport Sciences |
Degree: PhD |
Research interests: Exercise training, neuromuscular system, electrical stimulation |
E-mail: nicolas.babault@u-bourgogne.fr |
|
|
|
REFERENCES |
 Behm D.G., Bambury A., Cahill F., Power K. (2004) Effect of acute static stretching on force, balance, reaction time, and movement time. Medicine & Science in Sports & Exercise 36, 1397-1402.
|
 Behm D.G., Blazevich A.J., Kay A.D., McHugh M. (2016) Acute effects of muscle stretching on physical performance, range of motion, and injury incidence in healthy active individuals: a systematic review. Applied Physiology, Nutrition, and Metabolism 41, 1-11.
|
 Behm D.G., Chaouachi A. (2011) A review of the acute effects of static and dynamic stretching on performance. European Journal of Applied Physiology 111, 2633-2651.
|
 Bishop D (2003a) Warm up I: potential mechanisms and the effects of passive warm up on exercise performance. Sports Medicine (Auckland, NZ) 33, 439-454.
|
 Bishop D (2003b) Warm Up II. Sports Medicine 33, 483-498.
|
 Blazevich A.J., Cannavan D., Waugh C.M., Miller S.C., Thorlund J.B., Aagaard P., Kay A.D. (2014) Range of motion, neuromechanical, and architectural adaptations to plantar flexor stretch training in humans. Journal of Applied Physiology 117, 452-462.
|
 Bouvier T., Opplert J., Cometti C., Babault N. (2017) Acute effects of static stretching on muscle–tendon mechanics of quadriceps and plantar flexor muscles. European Journal of Applied Physiology 117, 1309-1315.
|
 Buchthal F., Kaiser E., Knappeis G.G. (1944) Elasticity, Viscosity and Plasticity in the Cross Striated Muscle Fibre. Acta Physiologica Scandinavica 8, 16-37.
|
 Budini F., Gallasch E., Christova M., Rafolt D., Rauscher A.B., Tilp M. (2017) One minute static stretch of plantar flexors transiently increases H reflex excitability and exerts no effect on corticospinal pathways. Experimental Physiology 102, 901-910.
|
 Cannavan D., Coleman D.R., Blazevich A.J. (2012) Lack of effect of moderate-duration static stretching on plantar flexor force production and series compliance. Clinical Biomechanics (Bristol, Avon) 27, 306-312.
|
 Cohen J (1988) Statistical power analysis for the behavioural sciences.
Statistical Power Analysis for the Behavioural Sciences L.
Erbaum. 14-68.
|
 Costa P.B., Herda T.J., Herda A.A., Cramer J.T. (2014) Effects of dynamic stretching on strength, muscle imbalance, and muscle activation. Medicine & Science in Sports & Exercise 46, 586-593.
|
 Cresswell A.G., Löscher W.N., Thorstensson A. (1995) Influence of gastrocnemius muscle length on triceps surae torque development and electromyographic activity in man. Experimental Brain Research 105, 283-290.
|
 Fletcher I.M. (2010) The effect of different dynamic stretch velocities on jump performance. European Journal of Applied Physiology 109, 491-498.
|
 Fletcher I.M., Monte-Colombo M.M. (2010) An investigation into the possible physiological mechanisms associated with changes in performance related to acute responses to different preactivity stretch modalities. Applied Physiology, Nutrition, and Metabolism 35, 27-34.
|
 Gajdosik R.L. (2001) Passive extensibility of skeletal muscle: review of the literature with clinical implications. Clinical Biomechanics 16, 87-101.
|
 Herda T.J., Costa P.B., Walter A.A., Ryan E.D., Cramer J.T. (2014) The time course of the effects of constant-angle and constant-torque stretching on the muscle-tendon unit. Scandinavian Journal of Medicine & Science in Sports 24, 62-67.
|
 Herda T.J., Costa P.B., Walter A.A., Ryan E.D., Hoge K.M., Kerksick C.M., Stout J.R., Cramer J.T. (2011) Effects of two modes of static stretching on muscle strength and stiffness. Medicine & Science in Sports & Exercise 43, 1777-1784.
|
 Herda T.J., Cramer J.T.J., Ryan E.E.D., McHugh M.P., Stout J.J.R. (2008) Acute effects of static versus dynamic stretching on isometric peak torque, electromyography, and mechanomyography of the biceps femoris muscle. Journal of Strength and Conditioning Research / National Strength & Conditioning Association 22, 809-817.
|
 Herda T.J., Herda N.D., Costa P.B., Walter-Herda A.A., Valdez A.M., Cramer J.T. (2012) The effects of dynamic stretching on the passive properties of the muscle-tendon unit. Journal of Sports Sciences 31, 479-487.
|
 Jaggers J.R., Swank A.M., Frost K.L., Lee C.D. (2008) The acute effects of dynamic and ballistic stretching on vertical jump height, force, and power. Journal of Strength and Conditioning Research / National Strength & Conditioning Association 22, 1844-1849.
|
 Kay A.D., Blazevich A.J. (2010) Concentric muscle contractions before static stretching minimize, but do not remove, stretch-induced force deficits. Journal of Applied Physiology (Bethesda, Md : 1985) 108, 637-645.
|
 Knudson, D. (2007) Fundamentals of Biomechanics. Springer US, Boston, MA
|
 Kubo K., Kanehisa H., Kawakami Y., Fukunaga T. (2001) Influence of static stretching on viscoelastic properties of human tendon structures in vivo. Journal of Applied Physiology 90, 520-527.
|
 Magnusson S.P., Simonsen E.B., Aagaard P., Boesen J., Johannsen F., Kjaer M. (1997) Determinants of musculoskeletal flexibility: viscoelastic properties, cross-sectional area, EMG and stretch tolerance. Scandinavian Journal of Medicine & Science in Sports 7, 195-202.
|
 Magnusson S.P., Simonsen E.B., Aagaard P., Dyhre-poulsen P., Mchugh M.P., Kjaer M., Ps A.M., Eb S., Aagaard P., Mchugh M., Mechanical K.M. (1996a) Mechanical and Physiological Responses to Stretching With and Without Preisometric Contraction in Human Skeletal Muscle. Archives of Physical Medicine and Rehabilitation 77, 373-378.
|
 Magnusson S.P., Simonsen E.B., Dyhre-Poulsen P., Aagaard P., Mohr T., Kjaer M. (1996b) Viscoelastic stress relaxation during static stretch in human skeletal muscle in the absence of EMG activity. Scandinavian Journal of Medicine & Science in Sports 6, 323-328.
|
 Maloney S.J., Turner A.N., Fletcher I.M. (2014) Ballistic exercise as a pre-activation stimulus : a review of the literature and practical applications. Sports Medicine 44, 1347-1359.
|
 McNair P.J., Dombroski E.W., Hewson D.J., Stanley S.N. (2000) Stretching at the ankle joint: viscoelastic responses to holds and continuous passive motion. Medicine & Science in Sports & Exercise 33, 354-358.
|
 Mizuno T., Matsumoto M., Umemura Y. (2013) Viscoelasticity of the muscle-tendon unit is returned more rapidly than range of motion after stretching. Scandinavian Journal of Medicine & Science in Sports 23, 23-30.
|
 Morse C.I., Degens H., Seynnes O.R., Maganaris C.N., Jones D.A. (2008) The acute effect of stretching on the passive stiffness of the human gastrocnemius muscle tendon unit. The Journal of Physiology 586, 97-106.
|
 Mutungi G., Ranatunga K.W. (1998) Temperature-dependent changes in the viscoelasticity of intact resting mammalian (rat) fast- and slow-twitch muscle fibres. The Journal of Physiology 508, 253-265.
|
 Noonan T.J., Best T.M., Seaber A. V., Garrett W.E. (1993) Thermal effects on skeletal muscle tensile behavior. The American Journal of Sports Medicine 21, 517-522.
|
 Nordez A., Cornu C., McNair P. (2006) Acute effects of static stretching on passive stiffness of the hamstring muscles calculated using different mathematical models. Clinical Biomechanics 21, 755-760.
|
 Nordez A., McNair P.J., Casari P., Cornu C. (2009) The effect of angular velocity and cycle on the dissipative properties of the knee during passive cyclic stretching: a matter of viscosity or solid friction. Clinical Biomechanics 24, 77-81.
|
 Opplert J., Babault N. (2018) Acute Effects of Dynamic Stretching on Muscle Flexibility and Performance: An Analysis of the Current Literature. Sports Medicine 48, 299-325.
|
 Opplert J., Genty J.-B., Babault N. (2016) Do Stretch Durations Affect Muscle Mechanical and Neurophysiological Properties?. International Journal of Sports Medicine 37, 673-679.
|
 Power K., Behm D., Cahill F., Carroll M., Young W. (2004) An acute bout of static stretching: effects on force and jumping performance. Medicine & Science in Sports & Exercise 36, 1389-1396.
|
 Ryan E.D., Beck T.W., Herda T.J., Hull H.R., Hartman M.J., Costa P.B., Defreitas J.M., Stout J.R., Cramer J.T. (2008b) The time course of musculotendinous stiffness responses following different durations of passive stretching. The Journal of Orthopaedic and Sports Physical Therapy 38, 632-639.
|
 Ryan E.D., Beck T.W., Herda T.J., Hull H.R., Hartman M.J., Stout J.R., Cramer J.T. (2008a) Do practical durations of stretching alter muscle strength? A dose-response study. Medicine & Science in Sports & Exercise 40, 1529-1537.
|
 Sale D.G. (2002) Postactivation Potentiation : Role in Human Performance. Exercise and Sport Sciences Reviews 30, 138-143.
|
 Taylor D.C., Dalton J.D., Seaber A.V., Garrett W.E. (1990) Viscoelastic properties of muscle-tendon units. The biomechanical effects of stretching. The American Journal of Sports Medicine 18, 300-309.
|
 Tillin N.A., Bishop D. (2009) Factors modulating post-activation potentiation and its effect on performance of subsequent explosive activities. Sports Medicine (Auckland, NZ) 39, 147-166.
|
 Trajano G.S., Nosaka K., Blazevich A.J. (2017) Neurophysiological mechanisms underpinning stretch-induced force loss. Sports Medicine 47, 1531-1541.
|
 Turki O., Chaouachi A., Drinkwater E.J., Chtara M., Chamari K., Amri M., Behm D.G. (2011) Ten minutes of dynamic stretching is sufficient to potentiate vertical jump performance characteristics. Journal of Strength and Conditioning Research / National Strength & Conditioning Association 25, 2453-2463.
|
 Yamaguchi T., Ishii K., Yamanaka M., Yasuda K. (2007) Acute effects of dynamic stretching exercise on power output during concentric dynamic constant external resistance leg extension. Journal of Strength and Conditioning Research / National Strength & Conditioning Association 21, 1238-1244.
|
 Yeh C.-Y., Tsai K.-H., Chen J.-J. (2005) Effects of prolonged muscle stretching with constant torque or constant angle on hypertonic calf muscles. Archives of Physical Medicine and Rehabilitation 86, 235-241.
|
 Young W.B., Behm D.G. (2002) Should static stretching be used during a warm-up for strength and power activities?. Strength and Conditioning Journal 24, 33-37.
|
|
|
|
|
|
|