|
|
|
ABSTRACT |
An affordable player monitoring solution could make the evaluation of external loading more accessible across multiple levels of football (soccer). The present study aimed to determine the accuracy of a newly designed and low-cost Global Positioning System (GPS) whilst performing match-specific movement patterns. Sixteen professional male football players (24 ± 3 years) were assigned a GPS device (TT01, Tracktics GmbH, Hofheim, Germany) and completed two experimental trials. In each trial, a continuous protocol including seven movements (sideways cornering, diagonal cornering, accelerating, decelerating, backwards jogging, shuttle running, and skipping) adding up to 500 m, was completed. Time-motion data was compared with criterion distance and velocity (photo-cell timing gates and radar). Validity was assessed through the standard error of the estimate (SEE) and reliability through the coefficient of variation (CV; both with 95% confidence limits). For the total distance covered during the protocol, the system was found to be valid (SEE = 3.1% [2.2; 5.8]) and reliable (intra-device CV = 2.0% [1.2; 7.6]). Similar results were found for velocity (SEE = 3.4% [2.6; 4.8], CV = 4.7% [3.2; 8.5]). In conclusion, the present GPS system, a low-cost solution, was found to be a valid and reliable tool for measuring physical loading during football-specific movements. |
Key words:
Soccer, team sports, external load, precision, motion analysis
|
Key
Points
- A newly designed and low-cost GPS system was found to provide reliable and valid data describing physical loading during football-specific movements.
- The system showed accurate measurements at higher running velocities, potentially due to different positioning of the devices on the body.
- The system relies on more affordable hardware components, offering a lower financial burden and making it available across a wider range of teams.
|
The use of Global Positioning Systems (GPS) within association football (soccer) has grown in recent years (Malone et al., 2017). GPS systems are regarded as an easy-to-administer tracking technology, which is not bound by instalment on a single pitch (Buchheit et al., 2014). Prior to July 2015, the use of this technology was restricted to training and friendly matches. It is only after this date, that the world governing body (Fédération Internationale de Football Association; FIFA) permitted the use of wearable technology during official matches. Consequently, this has led to an increase of investigations describing external player load during match play using GPS-monitoring (Martin-Garcia et al., 2018; Torreno et al., 2016). Most of these reports are based on data collected within professional teams (Trewin et al., 2017). This may possibly be due to financial restrictions of sub-elite teams, who may not be able to afford GPS systems. However, since a greater number of sub-elite players are active in comparison with elite players, a higher standard of (scientific) practice across multiple levels may further develop the sport. In other words, coaches across different levels could be offered objective information to improve match preparation and player selection, ultimately improving the quality of the players. Although technological developments have led to more advanced tracking solutions within elite environments, they also decreased the costs for basic hardware. This potentially allows for the collection of useful data with less advanced systems in team sport environments (Scott et al., 2016). In order to appropriately interpret the data from such systems, its accuracy has to be tested in ecologically valid situations, independent of the level of the intended users (Malone et al., 2017). This is of particular importance considering that errors were still found to become larger with increasing velocities, despite a greater overall precision for higher sampling rates (Rampinini et al., 2015; Scott et al., 2016). Subsequently, the aim of the present study was to determine the accuracy of a newly designed and low-cost GPS system. Both time-motion and reference data for distance and velocity were recorded during established football-specific movements.
DesignSimilar to previous studies (Coutts and Duffield, 2010; Hoppe et al., 2018), a continuous protocol including football-specific movements and two criterion measures was performed to analyse the validity and reliability of the collected time-motion data from a GPS system.
ParticipantsSixteen male football players (24 ± 3 years, 1.80 ± 0.03 m, 78 ± 4 kg) playing for a professional fourth division team, participated in the study and provided informed consent after the risks and benefits of the study had been explained. The study was ethically approved by the local Human Research Ethics Committee (Ärztekammer Saarland; ref: 23/17) and performed according to the Declaration of Helsinki.
ProceduresThe complete study consisted of three different trials. First, a familiarisation trial was performed, followed by two experimental trials to measure validity and reliability. The experimental trials were separated by 14 days, however normal training continued in between. The protocol consisted of seven types of movements over a 60 m course (see Figure 1), which proportionally simulated the activity previously observed during competitive football, both in style as well as the intensity of locomotion [see Table 1; (Bloomfield et al., 2007)]. The movements of the experimental trials were interspersed by short periods of static behaviour (5-10 s). These were included to enhance the ecologically validity of the protocol, as players are stationary for approximately 5% of playing time (Bloomfield et al., 2007). Each trial involved the continuous execution of the different movements and since some involved cornering or turns, a total distance of 500 m was covered per session (see Table 1). To ensure participants followed the planned course accurately, vertical pole gates and slalom poles were used which the participants had to cross and round as closely as possible.
MeasurementsThe GPS devices provided by the manufacturer (TT01, Tracktics GmbH, Hofheim, Germany; firmware version 1.7) collected positional data at a frequency of 5 Hz and triaxial accelerometer data at 200 Hz. Initially, the raw velocity data from the GPS-chip was computed onboard using two methods: positional differentiation and the Doppler-shift method. The final data describing distance, velocity and acceleration followed from the integration of input provided by all onboard components, such as the GPS-chip and accelerometer. These automated computations had been designed to continuously determine the most likely movement trajectory, depending on the quality of the available data. The GPS tracker was designed to be worn centred on the lower abdomen, within a specifically designed belt below the elastic band of the shorts (see Figure 2A and 2B). The belt moves the tracker in a forward-tilted position, optimising antenna functionality (see Figure 2C). Each participant was assigned a personal device, which was used throughout the study in order to minimise inter-unit variability. The devices were activated in advance, to ensure a sufficient satellite fix, indicated by a LED light on the devices, prior to each trial. The average number of satellites connected to the devices during the first trial was 13.3 ± 1.2 and for the second trial 13.5 ± 1.3. Furthermore, the average horizontal dilution of precision (HDOP), which describes the arrangement of the connected satellites across the sky, was 0.78 ± 0.05 and 0.75 ± 0.06 for the first and second trial, respectively. As it has been described that a connection to more than six satellites and HDOP values below 1 describe ideal conditions, the collected data was deemed suitable for analysis (Malone et al., 2017). As the GPS devices created encrypted data files, the time series of distance and velocity data were provided by the manufacturer, who was blinded to the testing protocol. These time series consisted of computed raw data, taking all input and metadata into account. No smoothing filters were applied at any time during the analyses within the current study. This data was then synchronised based on the time stamps of the criterion measures and the start of the GPS data was set for the first acceleration where a velocity >2 m·s-1 was reached after a 10 s period of static behaviour prior to the first section of the protocol (Hoppe et al., 2018). Actual distance as well as average and peak velocity were included as criterion measures and recorded using a measuring tape, single-beam timing gates (TC PhotoGate, Brower Timing, Draper, USA), and a hand-held radar gun (ATS-II, Stalker Sport, Richardson, USA). The timing gates were placed along the course to measure average velocity over intervals ranging from 20 to 50 m (Earp and Newton, 2012; Haugen et al., 2014). Peak velocity was measured for six players during sections C and D (see Figure 1; (Beato et al., 2018).
Statistical analysisOutcomes are displayed, where appropriately, as mean ± standard deviation (SD) and prior to analysis, all data was checked for normality using the Shapiro-Wilk test. Confidence limits (CL) were reported within square brackets. The standard error of the estimate (SEE) was calculated to determine the validity of the system for all different movements within the protocol (Petersen et al., 2009). The SEE is the standard deviation (reported along with 95% CL) of the %-difference between the GPS’s time-motion data and criterion measures. The percentage difference was reported as the bias of the devices. To determine the reliability of the GPS devices, both absolute errors (typical error; TE) and those expressed as a percentage (coefficient of variation; CV) were determined for distance and peak velocity (Jennings et al., 2010). The CV was rated qualitatively as good (<5%), moderate (5-10%), or poor (>10%) (Duthie et al., 2003; Scott et al., 2016). For the distance covered within the complete session, the true between-device SD was calculated using a linear mixed model. Within the model, trial was included as the fixed effect, whilst the device, device-by-trial interaction and the residual error were random effects. Furthermore, as the timing gates only provide average velocity, the GPS data was averaged for the corresponding section of the course and separated into four different speed zones: walking (<7.2 km·h-1), jogging (7.2-14.4 km·h-1), high-speed running (14.4-21.6 km·h-1), and very high-speed running (>21.6 km·h-1) (Dwyer and Gabbett, 2012). The same zones were also used to describe the intensity of the protocol, by separating the recorded GPS data. Both instances and distances per speed zone were based on a minimum duration of 0.6 s above the threshold speeds. Pearson correlations (r) were calculated to determine the validity of the speed values derived from the GPS and criterion measure, as well as the relationship between the error and average speed measures. The magnitude of correlation was assessed using the following thresholds: <0.1, trivial; 0.1 to 0.3, small; 0.3 to 0.5, moderate; 0.5 to 0.7, large; 0.7 to 0.9, very large; and 0.9 to 1.0, almost perfect (Hopkins et al., 2009). All statistical methods were conducted using the statistical software package SPSS (Version 24; SPSS Inc., Chicago, IL, USA).
The average distribution within the four speed zones throughout the protocol is presented in Table 2.
ValidityThe precision of the GPS system during all movements of the validation protocol is presented in Table 3. The SEE of the average velocity during the movements was found to be 5.4% [5.0; 5.9], with the average velocity measured by the timing gates and GPS indicating a significant and almost perfect correlation (r = 0.98 [0.97; 0.98], p < 0.01). The SEE for the speed zones was found to be 12.3% [10.5; 14.7] for walking, 9.3% [8.4; 10.4] for jogging, 6.0% [5.1; 7.3] for high-speed running, and 5.0% [3.4; 9.6] for very high-speed running. Here, a significant and almost perfect correlation between SEE and the different ranges of velocity was found, describing a greater accuracy at higher speeds (r = 0.96 [0.88; 0.99]; p = 0.04). No significant correlation was found between the average velocity of the seven sections and the absolute error (r = -0.29 [-0.86; 0.59], p = 0.26). The SEE of the peak speed was found to be 3.4% [2.6; 4.8], with no significant difference in the errors during movement C (maximal acceleration) and D (maximal deceleration; p = 0.39). Finally, a significant and very large correlation was found between peak speed measured by GPS and radar (r = 0.79 [0.57; 0.91], p < 0.01). The system was found to both over- and underestimate criterion distance for different movements within the protocol, resulting in a small overestimation bias for the complete session (0.8%). A systematic underestimation of criterion velocity was recorded, with an underestimating bias of 7.2%.
ReliabilityThe highest variation, as can be seen in Table 3, was found during skipping movements involving 180-degree turns while moving sideways. Collectively, the total distance of the session indicated a good reliability (<5%), the reliability for five of the drills was moderate (5-10% CV), whilst backward and skipping movements indicated poor reliability (>10% CV). Furthermore, a TE of 0.25 m·s-1 [0.17; 0.46] was found for the test-retest error within peak speed, leading to a CV of 4.7% [3.2; 8.5] of the error between the trials. A Bland-Altman analysis also revealed agreement within the intra-unit differences (see Figure 3).
Mixed modelAn observed 78.8% of the variance in total distance covered was due to the individual device. Since each player was assigned a specific device, the variance describes a players’ variation in the execution of the trial. A further 20.8% of variance was explained by the interaction between the device and trial. Finally, merely 0.4% was explained by random errors. The true between-device SD, the intercept of the model, was found to be 2.9% for the complete session, which translates to 14.5 m over the 500 m course.
The present study aimed to determine the accuracy of a newly designed low-cost GPS system. Time-motion data was gathered during a continuous protocol involving different movements specific to match-play in football and compared with two criterion measures (distance and velocity). The repeated execution of the protocol allowed for the calculation of the system’s reliability. Overall, good accuracy was found for the distance covered during the complete protocol, with a SEE of 3.1% [2.2; 5.8] for validity and a CV of 2.0% [1.2; 7.6] for intra-unit reliability. For peak velocity, despite a systematic underestimation, similar results were found, where a SEE of 3.4% [2.6; 4.8] and a CV of 4.7% [3.2; 8.5] indicate appropriate validity and reliability. Errors and variance increased when movements deviated from a straight line, however remained within previously reported ranges of acceptability (<10%; (Scott et al., 2016). Furthermore, variations in change of direction ability may lead to differences between individuals executing the identical course, leading to the largest portion of variance. However, the overall true between-device SD was found acceptable (2.9%). Interestingly, where previous studies have often found GPS systems to become less accurate with increasing velocities, the results of the current study indicated a small correlation between average velocity and the associated error (Coutts and Duffield, 2010; Jennings et al., 2010; Johnston et al., 2014). Relative to the velocity itself, the error was found to decrease with increasing average speeds over sections of the course. This effect was not due to changes of direction, which would decrease the average velocity, since no significant correlation between movement velocity and error was found. Additionally, the lowest accuracy was found for moving backwards in a straight line, whilst the greatest accuracy was found for shuttle running, which included four 180 degree turns. As such, high-speed movements, regardless of the direction of movement, may be confidently measured with the current GPS system. The significance of this finding lies with the notion that high-speed activities are considered highly important when analysing running behaviour in football (Carling et al., 2012; Faude et al., 2012). Whilst the GPS devices used in the previously mentioned studies are designed to be worn in between the scapulae for improved satellite reception, the devices used in the present study are worn on the lower abdomen (see Figure 1). A placement close to the centre of mass (COM) has been proposed as more sensitive to changes in human gait in comparison to a “standard” placement on the upper back (Barrett et al., 2016). This may be explained by biomechanics, which defines positional tracking as the displacement of an individual’s COM (Floor-Westerdijk et al., 2012). As such, the differences between the displacement and rotation of the COM and that of the shoulders whilst running are vastly different (Seay et al., 2011). With increasing velocities, arm swing becomes more pronounced and the greater displacement of the shoulders could increase noise for devices positioned in between the shoulder blades (Barrett et al., 2016). The same reasoning may explain the relatively limited accuracy of the current system when moving at lower velocities, sideways or backwards. When standing still, moving slowly, or not in a forward direction, movements of the abdomen and hips, unrelated to forward locomotion, might impact the accelerometer data. As introduced, this input plays a vital role in the final calculations of the time-motion data. Therefore, the positional data, otherwise relying on a relatively low sampling frequency of 5 Hz, may be greater affected. With increasing velocities, the movements around the COM will be predominately due to the locomotion itself, thereby possibly lowering the noise within the measurements and improving data accuracy. As such, this reduces the requirements for the hardware. For example, the current system features 5Hz GPS units in comparison with 15 Hz units in advanced systems used in elite environments. Consequently, the costs of the system can be minimised, increasing its availability across an array of competitive standards. To further identify the effect of wearing positions, being in between the shoulder blades or on the lower abdomen, devices should be tested more intensively. However, this proves difficult with commercial GPS systems, since the devices are designed to be worn due to the manufacturer’s specifications. The input from the GPS-chip and accelerometer are converged in such a way, that the final time-motion data is most accurate when the tracker is worn in its intended location. Therefore, wearing a second device in an unintended position and comparing the results would be unrelated to the accuracy and specificity of the system. Comparisons of performance data collected by systems worn in different positions should also be avoided until further studies have been performed regarding the effect of device placement. Because even when wearing two devices simultaneously close to their intended location, to calculate inter-unit variability (Rampinini et al., 2015), difficulties may arise. It has been found for different devices, that a certain distance should be present in between two devices, in order to exclude noise generated by the devices themselves (Hoppe et al., 2018). For the current system, it was deemed impossible to wear two devices on the designed location without affecting satellite reception, data or natural movements. It is because of these considerations, that the current study did not compare simultaneously worn units. However, the repeated use of the criterion measures provides an appropriate measure of intra-unit reliability (Beato et al., 2016). In order to allow for this intensified use of the criterion measures, the current study did not replicate previously performed protocols. Studies validating GPS systems for use in football have often performed a team sport running circuit (Bishop et al., 2001; Jennings et al., 2010). This protocol, however, only measures velocity over a short distance and thus, provides limited data on the variability of velocity. The criterion measures for velocity should, however, be discussed, as both single-beam timing systems and hand-held radar were used in the present study to measure average and peak velocity, respectively. For such timing systems, larger errors have been found for small inter-gate distances (Earp and Newton, 2012). Therefore, the gates were placed at least 20 m apart and hand-held radar was used to measure peak velocity whilst accelerating and decelerating (Haugen and Buchheit, 2016). This meant different references were used, both with their specific errors of measurement. A possible solution would be the use of a high-resolution multi-camera motion analysis system, capable of accurately measuring instant velocity, regardless of the running direction (Duffield et al., 2010). Moreover, the use of such a reference system could provide the validation of more complex motions, like jumps or accelerations and decelerations. This would be advantageous as such short and explosive actions are deemed highly influential on player load (Harper and Kiely, 2018). Accurately providing such information would further increase the impact of any tracking system. However, although desirable, this validation technique is costly and not easily accessible. Nonetheless, it could be attempted by future studies to use such a high-speed motion analysis video system to preferably validate a variety of tracking systems (Hoppe et al., 2018; Linke et al., 2018). Moreover, this may also lead to direct comparisons of the effect of wearing position, like the current abdominal solution and that of previously validated GPS systems, worn between the scapulae. Nevertheless, comprehensive data was collected through the protocol performed within the present study. The largest errors were found for sideways skipping and backwards jogging, which were found to be the least common types of locomotion during football matches (4.4% and 6.5% of time in motion, respectively; Bloomfield et al., 2007). Although better accuracy for all types of movements would be optimal, these errors do not seem to diminish the acceptable results for total distance and velocity.
Practical applicationsPrior to the implementation of a tracking system into practice, the characteristics of the data they collect should be understood, regardless of the population of players (Buchheit et al., 2014). The GPS system in the current study was found to provide accurate and valid movement data. These findings are of particular importance due to the devices being designed to be more accessible and available for sub-elite teams; extending its applicability across a broader spectrum of coaches and practitioners. Potentially improving the scientific standard across all levels of the sport, for both performance as well as medical applications, allowing for better monitoring of athletes. The accuracy of the current system, with a true-device SD of 2.9%, was deemed acceptable to indicate practically meaningful changes within the running load of players. For describing high-speed actions, considered highly important in football, the current system also shows to be a suitable tool, as acceptable accuracy was found for peak speed during sprinting and for average velocity within higher speed zones. Although no device-specific bias was found, the assignment of a specific device per player is advised, since no simultaneous tests were performed in the current study. Finally, practitioners should refrain from comparing training or match data from systems with different device placements, until further studies have been performed.
Taken together, the newly-designed GPS system used in the present study was shown to be sufficiently valid and reliable, and can thus be used with confidence for measuring running load in football. For the distance covered during the complete session, good validity, as well as intra-unit reliability was found. Peak velocity was found to be valid and reliable, since the systematic underestimation was determined unsubstantial. When changes in direction and style of locomotion were introduced, the accuracy decreased, however remained within previously published ranges of acceptability (<10%). Since the system relies on more affordable hardware components, it offers a lower financial burden which makes it available for sub-elite teams. This allows for a higher practical as well as scientific standard across multiple levels. Coaches and sport scientists may improve their monitoring and analyses, ultimately aiding the on-field performance. From a scientific standpoint, low-cost GPS devices like the currently validated systems may increase the numbers of studies performed, with greater sample sizes leading to a greater pool of data; providing novel insights into various aspects of football which elite teams may not be inclined to provide.
ACKNOWLEDGEMENTS |
No sources of funding were used to assist in the preparation of this article. Emiel Schulze is supported by a ‘Science and Health in Football’ scholarship funded by the DAAD (German Academic Exchange Service). The experiments comply with the current laws of the country in which they were performed. The authors have no conflict of interest to declare. The datasets generated during and/or analyzed during the current study are not publicly available, but are available from the corresponding author who was an organizer of the study. |
|
AUTHOR BIOGRAPHY |
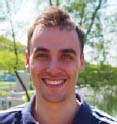 |
Emiel Schulze |
Employment: PhD student at Saarland University |
Degree: MSc |
Research interests: Analysis of physical, tactical and technical performance in elite football |
E-mail: e.schulze@uni-saarland.de |
|
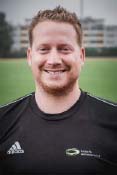 |
Ross Julian |
Employment: PhD student at Saarland University, lecturer at the Institute of Neuromotor Behavior and Exercise at Münster University and visiting research fellow at the University of Gloucestershire |
Degree: MSc |
Research interests: Physiology of female football, human performance during sports and exercise |
E-mail: julianr@uni-muenster.de |
|
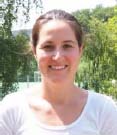 |
Sabrina Skorski |
Employment: Researcher and lecturer at Institute of Sports and Preventive Medicine at Saarland University |
Degree: PhD |
Research interests: Recovery and fatigue, pacing in endurance sports, physiology of swimming, sleep and athletic performance; |
E-mail: sabrina.forster@uni-saarland.de |
|
|
|
REFERENCES |
 Barrett S., Midgley A.W., Towlson C., Garrett A., Portas M., Lovell R. (2016) Within-Match PlayerLoad Patterns During a Simulated Soccer Match: Potential Implications for Unit Positioning and Fatigue Management. International Journal of Sports Physiology and Performance 11, 135-140.
|
 Beato M., Bartolini D., Ghia G., Zamparo P. (2016) Accuracy of a 10 Hz GPS Unit in Measuring Shuttle Velocity Performed at Different Speeds and Distances (5-20 M). Journal of Human Kinetics 54, 15-22.
|
 Beato M., Coratella G., Stiff A., Iacono A.D. (2018) The Validity and Between-Unit Variability of GNSS Units (STATSports Apex 10 and 18 Hz) for Measuring Distance and Peak Speed in Team Sports. Frontiers in Physiology 9, 1288.
|
 Bishop D., Spencer M., Duffield R., Lawrence S. (2001) The validity of a repeated sprint ability test. Journal of Science and Medicine in Sport 4, 19-29.
|
 Bloomfield J., Polman R., O’Donoghue P. (2007) Physical Demands of Different Positions in FA Premier League Soccer. Journal of Sports Science and Medicine 6, 63-70.
|
 Buchheit M., Allen A., Poon T.K., Modonutti M., Gregson W., Di Salvo V. (2014) Integrating different tracking systems in football: multiple camera semi-automatic system, local position measurement and GPS technologies. Journal of Sports Sciences 32, 1844-1857.
|
 Carling C., Le Gall F., Dupont G. (2012) Analysis of repeated high-intensity running performance in professional soccer. Journal of Sports Sciences 30, 325-336.
|
 Coutts A.J., Duffield R. (2010) Validity and reliability of GPS devices for measuring movement demands of team sports. Journal of Science and Medicine in Sport 13, 133-135.
|
 Duffield R., Reid M., Baker J., Spratford W. (2010) Accuracy and reliability of GPS devices for measurement of movement patterns in confined spaces for court-based sports. Journal of Science and Medicine in Sport 13, 523-525.
|
 Duthie G., Pyne D., Hooper S. (2003) The reliability of video based time motion analysis. Journal of Human Movement Studies 44, 259-271.
|
 Dwyer D.B., Gabbett T.J. (2012) Global positioning system data analysis: velocity ranges and a new definition of sprinting for field sport athletes. Journal of Strength and Conditioning Research 26, 818-824.
|
 Earp J.E., Newton R.U. (2012) Advances in electronic timing systems: considerations for selecting an appropriate timing system. Journal of Strength and Conditioning Research 26, 1245-1248.
|
 Faude O., Koch T., Meyer T. (2012) Straight sprinting is the most frequent action in goal situations in professional football. Journal of Sports Sciences 30, 625-631.
|
 Floor-Westerdijk M.J., Schepers H.M., Veltink P.H., van Asseldonk E.H., Buurke J.H. (2012) Use of inertial sensors for ambulatory assessment of center-of-mass displacements during walking. IEEE Transactions on Biomedical Engineering 59, 2080-2084.
|
 Harper D.J., Kiely J. (2018) Damaging nature of decelerations: Do we adequately prepare players?. BMJ Open Sport and Exercice Medicine 4, e000379.
|
 Haugen T., Buchheit M. (2016) Sprint Running Performance Monitoring: Methodological and Practical Considerations. Sports Medicine 46, 641-656.
|
 Haugen T.A., Tonnessen E., Svendsen I.S., Seiler S. (2014) Sprint time differences between single- and dual-beam timing systems. Journal of Strength and Conditioning Research 28, 2376-2379.
|
 Hopkins W.G., Marshall S.W., Batterham A.M., Hanin J. (2009) Progressive statistics for studies in sports medicine and exercise science. Medicine and Science in Sports and Exercise 41, 3-13.
|
 Hoppe M.W., Baumgart C., Polglaze T., Freiwald J. (2018) Validity and reliability of GPS and LPS for measuring distances covered and sprint mechanical properties in team sports. PLoS One 13, e0192708.
|
 Jennings D., Cormack S., Coutts A.J., Boyd L., Aughey R.J. (2010) The validity and reliability of GPS units for measuring distance in team sport specific running patterns. International Journal of Sports Physiology and Performance 5, 328-341.
|
 Johnston R.J., Watsford M.L., Kelly S.J., Pine M.J., Spurrs R.W. (2014) Validity and interunit reliability of 10 Hz and 15 Hz GPS units for assessing athlete movement demands. Journal of Strength and Conditioning Research 28, 1649-1655.
|
 Linke D., Link D., Lames M. (2018) Validation of electronic performance and tracking systems EPTS under field conditions. PLoS One 13, e0199519.
|
 Malone J.J., Lovell R., Varley M.C., Coutts A.J. (2017) Unpacking the Black Box: Applications and Considerations for Using GPS Devices in Sport. International Journal of Sports Physiology and Performance 12, S218-S226.
|
 Martin-Garcia A., Gomez Diaz A., Bradley P.S., Morera F., Casamichana D. (2018) Quantification of a Professional Football Team’s External Load Using a Microcycle Structure. Journal of Strength and Conditioning Research 32, 3511-3518.
|
 Petersen C., Pyne D., Portus M., Dawson B. (2009) Validity and reliability of GPS units to monitor cricket-specific movement patterns. International Journal of Sports Physiology and Performance 4, 381-393.
|
 Rampinini E., Alberti G., Fiorenza M., Riggio M., Sassi R., Borges T.O., Coutts A.J. (2015) Accuracy of GPS devices for measuring high-intensity running in field-based team sports. International Journal of Sports Medicine 36, 49-53.
|
 Scott M.T., Scott T.J., Kelly V.G. (2016) The Validity and Reliability of Global Positioning Systems in Team Sport: A Brief Review. Journal of Strength and Conditioning Research 30, 1470-1490.
|
 Seay J.F., Van Emmerik R.E., Hamill J. (2011) Low back pain status affects pelvis-trunk coordination and variability during walking and running. Clinical Biomechanics 26, 572-578.
|
 Torreno N., Munguia-Izquierdo D., Coutts A., de Villarreal E.S., Asian-Clemente J., Suarez-Arrones L. (2016) Relationship Between External and Internal Loads of Professional Soccer Players During Full Matches in Official Games Using Global Positioning Systems and Heart-Rate Technology. International Journal of Sports Physiology and Performance 11, 940-946.
|
 Trewin J.M.C., Varley M.C., Cronin J. (2017) The influence of situational and environmental factors on match-running in soccer: a systematic review. Science and Medicine in Football 1, 183-194.
|
|
|
|
|
|
|