|
|
|
ABSTRACT |
Self-massage using foam rollers, sticks, or balls has become a popular technique to enhance joint range of motion (ROM). Although increases are reported to be larger in females than males, the mechanisms of this observation are unclear. The present study aimed to investigate the effect of roller massage (RM) on ROM, passive tissue stiffness, and neurophysiological markers as a function of sex. Males (n = 15, 22.8 ± 2.9 yrs.) and females (n = 14, 21.1 ± 0.7 yrs.) performed three 60-second bouts of calf RM. Outcomes assessed pre-, and post-intervention included passive dorsiflexion (DF) ROM, passive tissue stiffness, passive torque, DF angle at the first stretch sensation, shear elastic modulus, and spinal excitability. DF ROM (+35.9 %), passive torque at DF ROM (+46.4 %), DF angle at first stretch sensation (+32.9 %), and pain pressure threshold (+25.2 %) increased in both groups (p<.05) with no differences between males and females (p > 0.05). No changes were observed for passive stiffness, shear elastic modulus, and spinal excitability (p > 0.05). Roller massage may increase ROM independently of sex, which, in the present study, could not be ascribed to alterations in passive stiffness or neurophysiological markers. Future studies may further elucidate the role of sensory alterations as possible factors driving RM-induced changes in flexibility. |
Key words:
Shear elastic modulus, dorsiflexion range of motion, stretch tolerance, passive torque, pain pressure threshold, H/M ratio
|
Key
Points
- We compared the effect of roller massage on range of motion, passive tissue stiffness, and neurophysiological markers as a function of sex.
- Roller massage intervention could increase range of motion and change in sensory, but could not change the passive tissue stiffness, and neurophysiological markers in both males and females.
- The increases in range of motion could only be related to changes in stretch perception (i.e., stretch tolerance) but not passive tissue stiffness, or spinal excitability.
|
Self-massage treatments using foam rollers, sticks, and balls have gained popularity in the sports, fitness, and rehabilitation sector. A plethora of studies demonstrated the effectiveness of related techniques to increase range of motion (ROM) (Krause et al., 2019; MacDonald et al., 2013; Wilke et al., 2019), reduce exercise-induced fatigue (de Benito et al., 2019; Fleckenstein et al., 2017), and alleviate muscle soreness/damage (Nakamura et al., 2020; Romero-Moraleda et al., 2019). Notably, in contrast to static stretching, rolling treatments do not induce declines in athletic performance parameters such as muscle strength, sprint speed, and jump height (Skinner et al., 2020; Wiewelhove et al., 2019), thus being of particular interest to athletes. A meta-analysis investigating the acute effects of foam rolling on ROM showed that it might be less effective in males than females (Wilke et al., 2020). However, to date, the mechanisms underlying this sex difference remain unclear. From a theoretical point of view, an increase in ROM after foam rolling could stem from both changes in passive soft tissue stiffness and neurophysiological alterations. Solid evidence suggests that foam rolling decreases the compressive stiffness of the anterior thigh (Baumgart et al., 2019; Wilke et al., 2019), Hamstring muscles (Morales-Artacho et al., 2017), and iliotibial tract (Mayer et al., 2019). Yet, some studies did not find significant changes in passive tensile stiffness of the anterior thigh (Krause et al., 2019) or compressive stiffness of the medial gastrocnemius muscle (MG) (Nakamura et al., 2021a), which suggests that foam rolling-induced ROM increases may also be due to sensory factors (i.e., altered stretch tolerance) (Behm and Wilke, 2019). Regarding neurophysiological changes, a previous study by Young et al. (2018) showed decreased H-reflex during roller massager (RM) application (Young et al., 2018). The authors proposed that the increases in ROM and pain pressure threshold (PPT) after the intervention could be attributed to inhibition at the spinal level. However, as the decreased H-reflex was restored immediately after rolling, this seems questionable. In view of the still uncertain relative contributions of tissue-specific, sensory and neural mechanisms to rolling-induced ROM increases and the unexplained effectiveness differences between male and female, the present study aimed to investigate the effect of RM on ROM, passive tissue stiffness, PPT, and neurophysiological response as a function of sex.
Experimental design and ethicsA randomized, two-armed parallel-group design with repeated measures was used to investigate sex differences in the response of the passive tissue properties of the plantar flexors and spinal excitability after RM. All participants were fully informed about the procedures and purpose of the study and provided with written informed consent. The Ethics Committee of the Niigata University of University of Health and Welfare, Niigata, Japan (Procedure #18304), approved the study, which complied with the Declaration of Helsinki requirements. Participants visited the laboratory three times. The first session was used for familiarization with the RM protocol and the passive ROM and PPT measurements, since all participants were not familiar with RM intervention. The following two sessions included the actual experiments. Here, in random order and with at least 72 hours of a wash-out, either passive properties (measurement order: dorsiflexion (DF) ROM, first stretch sensation (FSS), shear elastic modulus, and PPT) or spinal excitability (H and M wave) measurements were performed before and after the RM intervention. In all measurements, based on a previous study (Young et al., 2018), after the baseline measurement and before the RM intervention (PRE), a 10-minute break was included with the participants adopting a prone position. After this 10-minute break, the RM intervention with three 60-second bouts of RM and 30-second breaks in between was applied. Two minutes after the RM intervention, post measurements were conducted (POST).
ParticipantsRecruitment was performed using flyers and word of mouth. Fifteen males (mean ± SD: age, 22.8 ± 2.9 years; height, 1.70 ± 0.04 m; body mass, 63.4 ± 6.4 kg) and 14 females (age, 21.1±0.7 years; height, 1.60 ± 0.07 m; weight, 52.0 ± 9.3 kg) volunteered to participate. None of the enrolled individuals had a history of neuromuscular disease or musculoskeletal injury involving the lower extremities. All participants had not been involved in any regular resistance or flexibility training in the last 6 months. Sample size calculation was performed according the study of Czuppon et al. (2017) who reported a large magnitude of difference in ROM between the males and females. Hence, the sample size required for a two-way repeated measures analysis of variance (ANOVA) (effect size = 0.40 [large], α error = 0.05, and power = 0.80) using G* power 3.1 software (Heinrich Heine University, Düsseldorf, Germany) was more than 14 participants.
Roller massager (RM) interventionThe RM intervention was applied using a roller massager (Theraband, Akron, OH). Initially, participants were positioned prone on a treatment bed. An examiner moved the RM forward and backward on the muscle belly of the MG. The velocity of the applied strokes was controlled with a metronome set at 60 bpm. At each beep, the examiner either moved the massager one stroke up or down. The intensity was steered based on the participants’ feedback, targeting a discomfort of 7/10 on a numerical rating scale (0 representing no discomfort and 10 representing maximal discomfort), and the participants received three 60-second sets of RM with 30-second breaks between sets (Behm et al., 2020; Kiyono et al., 2020a).
Dorsiflexion range of motion and passive torqueDuring DF ROM measurements, the participants were in the prone position with a 0° knee angle. The measured foot was attached to a dynamometer’s (Biodex System 3.0, Biodex Medical Systems Inc., Shirley, NY, USA) footplate. Using the continuous passive motion function, the device moved the ankle into passive dorsiflexion at a speed of 5°/s, starting from a 30° plantarflexion (i.e., anatomical neutral position was 0°). The measurements were performed until the participants felt discomfort, stopping the dynamometer by activating the safety trigger (Kiyono et al., 2020a). The angle achieved at this point was defined as the DF ROM, and the passive torque at DF ROM was documented as the sensory perception index. In addition, we calculated the passive stiffness of the muscle-tendon unit as the change in the passive torque from the neutral ankle position (0°) to the least dorsiflexion angle before and after the RM intervention divided by the change of the joint angle (Konrad et al., 2017). Based on previous studies (Konrad and Tilp, 2014), two warm-up cycles were performed PRE-measurement, avoiding a conditioning effect of the passive dorsiflexion test on the passive stiffness. After the two warm-up cycles, DF ROM and passive torque were measured twice, utilizing the averages for further analysis. We confirmed that there was no heel raise during the DF ROM measurements during the familiarization cycles and measurements. Throughout the passive dorsiflexion test, the participants were requested to relax completely, not generating any voluntary resistance to the applied stretch. The absence of voluntary contraction was verified by monitoring electromyography (see below).
Joint angle at first stretch sensationThe FSS angle was defined as the first self-perceived stretch sensation during the DF ROM measurements. The participants were instructed to stop the passive movement at the angle of the FSS using the safety trigger (Krause et al., 2019). FSS angle was measured twice, using the average value for further analysis.
Shear elastic modulus of the medial gastrocnemius (MG)The MG shear elastic modulus was measured by means of an ultrasonic shear wave elastography imaging device (Aixplorer Supersonic Imagine, Aix-en-Provence, France) with a SL10-2 linear probe. Measurements were taken at an anatomical neutral ankle position (0°), which is the same position as the DF ROM measurements. The MG shear elastic modulus was assessed at 30% of the lower leg length from the popliteal crease to the lateral malleolus near the point of the lower leg’s maximal cross-sectional area (Nakamura et al., 2019). The size of the region of interest was 10 × 20 mm2 near the center of the MG, with a 5-mm-diameter-circle analysis area at the center of the region (Saeki et al., 2019). Elastography images of the MG long axis were obtained twice. Based on previous studies (Kiyono et al., 2020a; Nakamura et al., 2019), the shear elastic modulus was calculated by dividing the obtained Young’s modulus by 3, while obtaining the ultrasound measurements twice and using the average shear elastic modulus value for analysis.
Pain pressure threshold (PPT)For the PPT measurements, we used an algometer (NEUTONE TAM-22 (BT10); TRY ALL, Chiba, Japan). The participants adopted a prone position on the treatment bed, again similar to the DF ROM measurements, with the ankle joint at 20° plantarflexion. The metal rod of the algometer was used to compress the soft tissue in the measurement area, which is the same location with shear elastic modulus assessment. The participants were instructed to immediately press a trigger when pain rather than just pressure was felt, with the value (kilograms per square centimeter) from the device, at this time point, corresponding to the PPT. We used the mean value of the three repeated measurements with a 30-second interval for data analysis (Kim and Lee, 2018; Naderi et al., 2020).
Electromyography and spinal cord motoneuron excitability assessmentThe electromyographic activity of the soleus muscle was recorded using self-adhesive electrodes with a recording surface (Ambu, Blue Sensor N). Prior to the measurements, the skin was cleaned with alcohol, improving conductivity. Electrodes were placed on the soleus according to the SENIAM recommendations (Hermens et al., 2000), with the ground electrode placed between the electrical stimulation and the surface electromyogram electrodes. Electromyographic activity was filtered with a 10–1000 Hz band-pass filter (Fa-DL-720-140, 4Assist, Tokyo, Japan), the common mode rejection ratios>110 dB, and 5.1Mθ© input impedances. The electromyographic activity was digitally stored (10 kHz sampling rate) on a personal computer for offline analysis performed using PowerLab 8/30 and LabChart 7 (AD Instruments, Colorado Springs, CO, USA). In the passive dorsiflexion test, we monitored the soleus muscle activity to verify inactivity. We induced nerve stimulation using a constant-current stimulator (Isolator SS-104 J: Nihon Kohden Corporation) targeting the tibial nerve using 1-ms-square pulses. Based on previous research (Blazevich et al., 2014; Kiyono et al., 2020b; Stutzig and Siebert, 2017), the tibial nerve, selectively stimulated in a monopolar manner, induced soleus H-reflex and M waves. The anode was placed on the patella, and the cathode on the popliteal fossa overlying the nerve positioned to provide the greatest H wave amplitude at the smallest stimulus intensity, which was identified by stimulating the different skin surface sites with relatively low currents. Electrodes were attached to the skin using a surgical tape, preventing unwanted movement during testing. The H/M recruitment curves were assessed before and after the RM sessions. At the beginning of each, the H-reflex and M wave recruitment curves were measured under resting conditions to determine the H-reflex (Hmax) and M wave (Mmax) maximum amplitudes. The stimulations were increased by 0.5 milliamps every 10 s until Mmax was reached, retaining the corresponding intensity for all stimulations for analysis. The H/M ratio, a spinal excitability index, was calculated from the measured H and M waves (Blazevich et al., 2014; Stutzig and Siebert, 2017).
Statistical analysisDescriptive data are presented as means ± SD. The normal distribution of all variables was tested by means of the Shapiro-Wilk test. Comparisons of PRE values between males and females were determined by the unpaired t-test. In case of significant differences, relative changes (%) were used in the following steps (Mann-Whitney-U-test). If there were no significant differences between PRE values, a split-plot 2x2 ANOVA [time (PRE vs. POST) × gender [male vs. female]) was used to investigate the interactions and main effects. If there was a main effect for time, post hoc paired t-tests with Bonferroni correction were performed to reveal PRE-POST changes in each group. The effect size was calculated as a difference in the mean value between PRE and POST divided by the pooled standard deviation (SD) with effect sizes of 0.00–0.19, 0.20–0.49, 0.50–0.79, and ≥0.80 being considered trivial, small, moderate, and large, respectively (Cohen, 1988). Finally, we investigated the correlation between changes in DF ROM and in each variable using Spearman’s rank correlation coefficient. The significance threshold was set to α=0.05. We used SPSS version 24.0 (IBM Corp., Armonk, NY, USA) for statistical analyses.
Comparison of the pre-intervention values between males and femalesAll values are shown in Table 1. Unpaired t-tests showed that passive stiffness in males was significantly higher than that in females (p < 0.01, d = 1.12). No differences were found for the remaining variables.
Changes in all variables after the RM interventionThe Mann-Whitney-U-test did not yield a significant difference in the relative pre-post changes between sexes regarding passive stiffness (male: –1.2 ± 13.0% vs. female: 5.2 ± 13.5%, p=0.73, d = 0.48). Similarly, the split-plot ANOVAs revealed no significant interaction effects for the other variables (Table 1). However, main effects of time were found regarding DF ROM, passive torque at DF ROM, FSS, and PPT. Post-hoc testing showed that DF ROM, passive torque at DF ROM, FSS, and PPT increased in both males and females. No main effects occurred for the MG shear elastic modulus and H/M ratio.
The relationship between the changes in DF ROM and all variablesSignificant correlations were observed between the changes in DF ROM and passive torque at DF ROM in both, males and females (Figure 1A: rs = 0.779, p < 0.01; Figure 1B: rs = 0.713, p< 0.01, respectively). Conversely, there were no significant correlations with passive stiffness (male: rs = –0.101, p = 0.60; female: rs = 0.02, p = 0.95), FSS (male: rs = 0.432, p = 0.11; female: rs = 0.411; p = 0.13), PPT (male: rs = 0.381, p = 0.16; female: rs = –0.202, p = 0.47), MG shear elastic modulus (male: rs = 0.107, p = 0.70; female: rs = 0.193, p = 0.49), and H/M ratio (male: rs = 0.464, p = 0.08; rs = –0.275; p = 0.32).
To the best of our knowledge, our study is the first to investigate the mechanical, sensory, and neurophysiological correlates of sex differences in ROM increases following RM. Our RM intervention enhanced ROM and PPT while modifying stretch sensation. However, interestingly, there were no sex differences in changes after the RM intervention. Moreover, there were no significant changes in passive stiffness and spinal excitability. Finally, there were high positive correlations between the changes in DF ROM and passive torque at DF ROM in both males and females, suggesting that increased ROM may rather stem from altered sensory perception than changes in passive tissue stiffness or spinal excitability. As indicated, the results of the present study revealed increased DF ROM after a 180-second (three sets of 60-second) RM intervention, which is generally in accordance with previous works (Krause et al., 2019; Nakamura et al., 2021a; Wilke et al., 2019). However, in contrast to a recent meta-analysis (Wilke et al. 2020), there were no significant sex differences. The reason for the discrepancy between the results of this study and those of previous works is obscure. However, there were no significant interaction effects of the RM intervention regarding the passive tissue properties, pain sensory perception, and spinal excitability. Thus, in the case of the calf muscle, there might be no sex difference in response to the RM intervention. In their analysis, Wilke et al. (2020) pointed out that the treatment response of foam rolling interventions varies between muscles, and the effect sizes were lower for the calf (ES = 0.43) than for the thigh muscles (hamstring, ES = 1.0; quadriceps, ES = 0.83) (Wilke et al., 2020). It may hence be that sex differences do only manifest in those regions, and future research in this area is warranted. In addition to the changes in DF ROM, the present study found significant alterations of the passive torque at DF ROM, FSS, and PPT, which is consistent with the available evidence (Aboodarda et al., 2015; Cavanaugh et al., 2017; Cheatham and Stull, 2019; Krause et al., 2019; Nakamura et al., 2021a). Moreover, there were significant positive correlations between the changes in DF ROM and passive torque at DF ROM. These results show that changes in stretch perception could represent an overlooked contributor to ROM increases. The previous study showed that PPT was increased after a foam rolling intervention on both the intervention and non-intervention sides and suggested global pain modulatory inhibition of pain after a foam rolling intervention (Aboodarda et al., 2015). Hence, our results strengthen the assumption that foam rolling modifies sensory perception, e.g., via involvement of the gate control theory, parasympathetic hyperactivity, and diffuse noxious inhibitory control (Behm and Wilke, 2019). Although the exact mechanism in the present study is unknown, the aforementioned processes might also have caused the changes in FSS and PPT. Foam rolling and the RM intervention have been shown to modify the myofascial viscoelastic properties by mechanisms, such as viscosity reductions, loosening of myofascial restrictions, fluid changes, and cellular responses (Cheatham and Stull, 2019; Kelly and Beardsley, 2016). Our study did not reveal significant changes in passive stiffness and shear elastic modulus, which is consistent with some of the previous studies (Krause et al., 2019; Nakamura et al., 2021a; Yoshimura et al., 2020). In contrast, other earlier trials showed that foam rolling decreases the passive stiffness of the anterior thigh (Wilke et al., 2019) or the shear elastic modulus of the hamstring (Morales-Artacho et al., 2017). Again, these findings may be due to the treated body region (thigh vs. calf). Besides mechanical alterations in the soft tissue, previous research has also reported changes in spinal excitability (Grabow et al., 2018). A previous trial investigated RM effects on the H-reflex, detecting a decrease during the intervention but an immediate return to baseline after its end (Young et al., 2018). As shown, the present study did not detect a change in the H/M ratio 2 minutes after the RM intervention. The discrepancy may be ascribed to the timing of intervention and measurements (during vs. post-intervention). Therefore, RM-induced changes of spinal excitability may occur during the intervention but cease immediately after rolling. In summary, the change in inhibition at the spinal level could not be attributed to a ROM increase after the RM intervention. Some methodological aspects merit consideration. With 29 participants, our study had a rather small sample size and may have lacked statistical power. For instance, in some variables, descriptive increases amounted to up to 10% but failed to meet the significance threshold despite medium effect sizes. It hence needs to be underlined that further confirmatory trials are needed to verify our exploratory assumptions. Another issue relates to the type of rolling. We used an examiner to apply the soft tissue massage treatment. In clinical practice, most athletes will actively use foam rollers on their own. Therefore, comparative studies examining the validity of externally and self-applied pressure are urgent needs for the future. Also, we measured DF ROM with extended knees and muscle stiffness of the MG but H/M ratio at the soleus muscle. Future studies should consider creating congruency in this regard. Finally, there was no control condition (no intervention condition) in this study. The possibility that the PRE-measurement stimulation could contribute to the changes in DF ROM and stretch tolerance. However, our previous studies confirmed that these variables were highly reliable (Nakamura et al., 2021b; Sato et al., 2020), and the changes in these variables could be contributed by RM intervention.
The present study investigated the effect of RM on DF ROM, passive torque, FSS, shear elastic modulus, PPT, and H/M ratio, comparing the effects between males and females. Our results showed that DF ROM, passive torque at DF ROM, FSS, and PPT increased after the RM intervention independent of sex. The increases in DF ROM could only be related to changes in stretch perception (i.e., stretch tolerance) but not passive stiffness, FSS, PPT, or spinal excitability. Further research delineating mechanisms of foam rolling as well as their relative contribution in males and females is therefore warranted.
ACKNOWLEDGEMENTS |
The authors would like to thank Enago () for editorial assistance with the manuscript. This work was supported by JSPS KAKENHI with grant number 19K19890 (Masatoshi Nakamura), and the Austrian Science Fund (FWF) project P 32078-B (Andreas Konrad). The experiments comply with the current laws of the country in which they were performed. The authors have no conflict of interest to declare. The datasets generated during and/or analyzed during the current study are not publicly available, but are available from the corresponding author who was an organizer of the study. |
|
AUTHOR BIOGRAPHY |
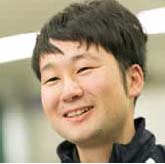 |
Masatoshi Nakamura |
Employment: Lecture, Institute for Human Movement and Medical Sciences, Niigata University of Health and Welfare, Niigata, Niigata, Japan |
Degree: PhD |
Research interests: Physical therapy, stretching, exercise physiology, flexibility |
E-mail: masatoshi-nakamura@nuhw.ac.jp |
|
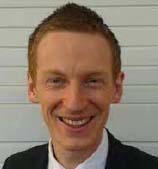 |
Andreas Konrad |
Employment: Institute of Human Movement Science, Sport and Health, University of Graz |
Degree: PhD, MSc |
Research interests: Biomechanics, muscle performance, training science, muscle-tendon-unit, soccer science |
E-mail: andreas.konrad@uni-graz.at |
|
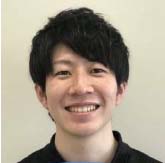 |
Kiyono Ryosuke |
Employment: Institute for Human Movement and Medical Sciences, Niigata University of Health and Welfare, Niigata, Niigata, Japan |
Degree: MSc |
Research interests: Physical therapy, foam rolling, flexibility, exercise physiology |
E-mail: hpm19005@nuhw.ac.jp |
|
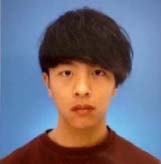 |
Shigeru Sato |
Employment: Institute for Human Movement and Medical Sciences, Niigata University of Health and Welfare, Niigata, Niigata, Japan |
Degree: MSc, PhD student |
Research interests: Physical therapy, stretching, flexibility, resistance training |
E-mail: hpm19006@nuhw.ac.jp |
|
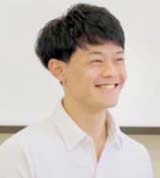 |
Kaoru Yahata |
Employment: Institute for Human Movement and Medical Sciences, Niigata University of Health and Welfare, Niigata, Niigata, Japan |
Degree: BSc, MSc student |
Research interests: Physical therapy, stretching, flexibility, delayed onset muscle soreness |
E-mail: hpm20011@nuhw.ac.jp |
|
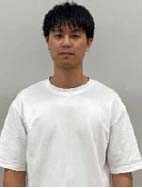 |
Riku Yoshida |
Employment: Institute for Human Movement and Medical Sciences, Niigata University of Health and Welfare, Niigata, Niigata, Japan |
Degree: BSc, MSc student |
Research interests: Physical therapy, resistance training, cross-education |
E-mail: hpm21017@nuhw.ac.jp |
|
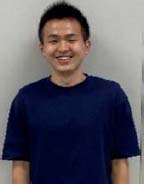 |
Yuta Murakami |
Employment: Department of Physical Therapy, Niigata University of Health and Welfare, Niigata, Niigata, Japan |
Degree: BSc student |
Research interests: Physical therapy, stretching, training |
E-mail: hpm21017@nuhw.ac.jp |
|
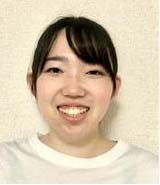 |
Futaba Sanuki |
Employment: Department of Physical Therapy, Niigata University of Health and Welfare, Niigata, Niigata, Japan |
Degree: BSc student |
Research interests: Physical therapy, stretching, thermal therapy |
E-mail: hpm21017@nuhw.ac.jp |
|
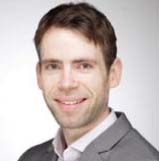 |
Jan Wilke |
Employment: Institute of Occupational, Social and Environmental Medicine, Goethe University Frankfurt, Germany |
Degree: PhD |
Research interests: Strength and Conditioning, Exercise & Neurocognition, Biomechanics of the Locomotor System |
E-mail: wilke@sport.uni-frankfurt.de |
|
|
|
REFERENCES |
 Aboodarda S.J., Spence A.J., Button D.C. (2015) Pain pressure threshold of a muscle tender spot increases following local and non-local rolling massage. BioMed Central Musculoskelet Disord 16, 265. Crossref
|
 Baumgart C., Freiwald J., Kühnemann M., Hotfiel T., Hüttel M., Hoppe M.W. (2019) Foam Rolling of the Calf and Anterior Thigh: Biomechanical Loads and Acute Effects on Vertical Jump Height and Muscle Stiffness. Sports (Basel) 7, 27. Crossref
|
 Behm D.G., Alizadeh S., Hadjizadeh Anvar S., Mahmoud M.M.I., Ramsay E., Hanlon C., Cheatham S. (2020) Foam Rolling Prescription: A Clinical Commentary. The Journal of Strength & Conditioning Research 34, 3301-3308. Crossref
|
 Behm D.G., Wilke J. (2019) Do Self-Myofascial Release Devices Release Myofascia? Rolling Mechanisms: A Narrative Review. Sports Medicine 49, 1173-1181. Crossref
|
 Blazevich A.J., Cannavan D., Waugh C.M., Miller S.C., Thorlund J.B., Aagaard P., Kay A.D. (2014) Range of motion, neuromechanical, and architectural adaptations to plantar flexor stretch training in humans. Journal of Applied Physiology 117, 452-462. Crossref
|
 Cavanaugh M.T., Döweling A., Young J.D., Quigley P.J., Hodgson D.D., Whitten J.H., Reid J.C., Aboodarda S.J., Behm D.G. (2017) An acute session of roller massage prolongs voluntary torque development and diminishes evoked pain. European Journal of Applied Physiology 117, 109-117. Crossref
|
 Cheatham S.W., Stull K.R. (2019) Roller massage: Comparison of three different surface type pattern foam rollers on passive knee range of motion and pain perception. Journal of Bodywork and Movement Therapies 23, 555-560. Crossref
|
 Cohen, J. (1988) Statistical power analysis for the behavioral sciences.,Hillsdale, Routledge.
|
 Czuppon S., Prather H., Hunt D.M., Steger-May K., Bloom N.J., Clohisy J.C., Larsen R., Harris-Hayes M. (2017) Gender-Dependent Differences in Hip Range of Motion and Impingement Testing in Asymptomatic College Freshman Athletes. American Academy of Physical Medicine and Rehabilitation 9, 660-667. Crossref
|
 de Benito A.M., Valldecabres R., Ceca D., Richards J., Barrachina Igual J., Pablos A. (2019) Effect of vibration vs non-vibration foam rolling techniques on flexibility, dynamic balance and perceived joint stability after fatigue. PeerJ 7, e8000. Crossref
|
 Fleckenstein J., Wilke J., Vogt L., Banzer W. (2017) Preventive and Regenerative Foam Rolling are Equally Effective in Reducing Fatigue-Related Impairments of Muscle Function following Exercise. Journal of Sports Science and Medicine 16, 474-479. Pubmed
|
 Grabow L., Young J.D., Alcock L.R., Quigley P.J., Byrne J.M., Granacher U., Škarabot J., Behm D.G. (2018) Higher Quadriceps Roller Massage Forces Do Not Amplify Range-of-Motion Increases nor Impair Strength and Jump Performance. Journal of Strength & Conditioning Research 32, 3059-3069. Crossref
|
 Hermens H.J., Freriks B., Disselhorst-Klug C., Rau G. (2000) Development of recommendations for SEMG sensors and sensor placement procedures. Journal of Electromyography and Kinesiology 10, 361-374. Crossref
|
 Kelly S., Beardsley C. (2016) Specific and cross-over effects of foam rolling on ankle dorsiflexion range of motion. International Journal of Sports Physical Therapy 11, 544-551.
|
 Kim S.J., Lee J.H. (2018) Effects of sternocleidomastoid muscle and suboccipital muscle soft tissue release on muscle hardness and pressure pain of the sternocleidomastoid muscle and upper trapezius muscle in smartphone users with latent trigger points. Medicine (Baltimore) 97, e12133. Crossref
|
 Kiyono R., Onuma R., Yasaka K., Sato S., Yahata K., Nakamura M. (2020a) Effects of 5-Week Foam Rolling Intervention on Range of Motion and Muscle Stiffness. Journal of Strength & Conditioning Research. Crossref
|
 Kiyono R., Sato S., Inaba K., Yahata K., Nakamura M. (2020b) Time course of changes in range of motion, muscle shear elastic modulus, spinal excitability, and muscle temperature during superficial icing. Sport Sciences for Health 17, 341-346. Crossref
|
 Konrad A., Stafilidis S., Tilp M. (2017) Effects of acute static, ballistic, and PNF stretching exercise on the muscle and tendon tissue properties. Scandinavian Journal of Medicine & Science in Sports 27, 1070-1080. Crossref
|
 Konrad A., Tilp M. (2014) Effects of ballistic stretching training on the properties of human muscle and tendon structures. Journal of Applied Physiology 117, 29-35. Crossref
|
 Krause F., Wilke J., Niederer D., Vogt L., Banzer W. (2019) Acute effects of foam rolling on passive stiffness, stretch sensation and fascial sliding: A randomized controlled trial. Human Movement Science 67, 102514. Crossref
|
 MacDonald G.Z., Penney M.D., Mullaley M.E., Cuconato A.L., Drake C.D., Behm D.G., Button D.C. (2013) An acute bout of self-myofascial release increases range of motion without a subsequent decrease in muscle activation or force. Journal of Strength & Conditioning Research 27, 812-821. Crossref
|
 Mayer I., Hoppe M.W., Freiwald J., Heiss R., Engelhardt M., Grim C., Lutter C., Huettel M., Forst R., Hotfiel T. (2019) Different Effects of Foam Rolling on Passive Tissue Stiffness in Experienced and Nonexperienced Athletes. Journal of Sport Rehabilitation 29, 926-933. Crossref
|
 Morales-Artacho A.J., Lacourpaille L., Guilhem G. (2017) Effects of warm-up on hamstring muscles stiffness: Cycling vs foam rolling. Scandinavian Journal of Medicine & Science in Sports 27, 1959-1969. Crossref
|
 Naderi A., Rezvani M.H., Degens H. (2020) Foam Rolling and Muscle and Joint Proprioception After Exercise-Induced Muscle Damage. Journal of Athletic Training 55, 58-64. Crossref
|
 Nakamura M., Kiyono R., Sato S., Yahata K., Fukaya T., Nishishita S., Konrad A. (2021a) The Associations between Rapid Strength Development and Muscle Stiffness in Older Population. Healthcare (Basel) 9, 80. Crossref
|
 Nakamura M., Sato S., Kiyono R., Takahashi N., Yoshida T. (2019) Effect of Rest Duration Between Static Stretching on Passive Stiffness of Medial Gastrocnemius Muscle in vivo. Journal of Sport Rehabilitation 29, 578-582. Crossref
|
 Nakamura M., Sato S., Kiyono R., Yahata K., Yoshida R., Fukaya T., Konrad A. (2021b) Comparison of the Acute Effects of Hold-Relax and Static Stretching among Older Adults. Biology) 10, 126. Crossref
|
 Nakamura M., Yasaka K., Kiyono R., Onuma R., Yahata K., Sato S., Konrad A. (2020) The Acute Effect of Foam Rolling on Eccentrically-Induced Muscle Damage. International Journal of Environmental Research and Public Health 18, 75-. Crossref
|
 Romero-Moraleda B., González-García J., Cuéllar-Rayo Á., Balsalobre-Fernández C., Muñoz-García D., Morencos E. (2019) Effects of Vibration and Non-Vibration Foam Rolling on Recovery after Exercise with Induced Muscle Damage. Journal of Sports Science and Medicine 18, 172-180. Pubmed
|
 Saeki J., Ikezoe T., Yoshimi S., Nakamura M., Ichihashi N. (2019) Menstrual cycle variation and gender difference in muscle stiffness of triceps surae. Clinical Biomechanics 61, 222-226. Crossref
|
 Sato S., Kiyono R., Takahashi N., Yoshida T., Takeuchi K., Nakamura M. (2020) The acute and prolonged effects of 20-s static stretching on muscle strength and shear elastic modulus. Plos One 15, e0228583. Crossref
|
 Skinner B., Moss R., Hammond L. (2020) A systematic review and meta-analysis of the effects of foam rolling on range of motion, recovery and markers of athletic performance. Journal of Bodywork and Movement Therapies 24, 105-122. Crossref
|
 Stutzig N., Siebert T. (2017) Assessment of the H-reflex at two contraction levels before and after fatigue. Scandinavian Journal of Medicine & Science in Sports 27, 399-407. Crossref
|
 Wiewelhove T., Döweling A., Schneider C., Hottenrott L., Meyer T., Kellmann M., Pfeiffer M., Ferrauti A. (2019) A Meta-Analysis of the Effects of Foam Rolling on Performance and Recovery. Frontiers Physiology 10, 376. Crossref
|
 Wilke J., Müller A.L., Giesche F., Power G., Ahmedi H., Behm D.G. (2020) Acute Effects of Foam Rolling on Range of Motion in Healthy Adults: A Systematic Review with Multilevel Meta-analysis. Sports Medicine 50, 387-402. Crossref
|
 Wilke J., Niemeyer P., Niederer D., Schleip R., Banzer W. (2019) Influence of Foam Rolling Velocity on Knee Range of Motion and Tissue Stiffness: A Randomized, Controlled Crossover Trial. Journal of Sport Rehabilitation 28, 711-715. Crossref
|
 Yoshimura A., Schleip R., Hirose N. (2020) Effects of Self-Massage Using a Foam Roller on Ankle Range of Motion and Gastrocnemius Fascicle Length and Muscle Hardness: A Pilot Study. Journal of Sport Rehabilitation 29, 1171-1178. Crossref
|
 Young J.D., Spence A.J., Behm D.G. (2018) Roller massage decreases spinal excitability to the soleus. Journal of Applied Physiology 124, 950-959. Crossref
|
|
|
|
|
|
|