|
|
|
ABSTRACT |
The dynamic strength index (DSI) is calculated as the ratio between countermovement jump (CMJ) peak force and isometric mid-thigh pull (IMTP) peak force and is said to inform whether ballistic or strength training is warranted for a given athlete. This study assessed the impact of an individualized in-season resistance training program, guided by DSI on basketball players’ physical performance. Forty-three elite players (19.4 ± 2.9 years; 1.97 ± 0.08 cm; 89.1 ± 9.5 kg) were divided into an intervention group (IG) (27 players) and a control group (CG) (16 players). The IG was further split based on DSI into a ballistic group (DSI ≤ 0.90, 11 players) and a strength group (DSI > 0.90, 16 players). Over five weeks, participants underwent two weekly resistance sessions, with the IG following a DSI-based program and the CG a standard program. Performance was measured pre- and post-intervention through 20-m sprints, 505 change of direction test, CMJ, and IMTP. There were statistically significant improvements in the IG, notably in sprint times (η2 = 0.12-0.21, p < 0.05) and 505 test (η2 = 0.15-0.16, p < 0.05), predominantly in the strength group. The CG’s performance was either unchanged or declined for different variables. Our results suggest that DSI-guided training effectively enhances basketball players’ physical performance within a competitive season. |
Key words:
Athletes, performance, jumps, strength, power, testing
|
Key
Points
- Evaluating DSI and programming based on DSI values is an efficient way to enhance basketball players’ physical performance within a competitive season.
- Individualized training consisted of three exercises of three sets, performed twice a week is sufficient to improve (or prevent a decrease in) the physical performance of basketball players during the basketball season.
- An average DSI value of 0.9 indicates that basketball players are largely velocity/ballistic dominant athletes, thus strength training may be the preferable option during the basketball season for improving physical performance if individualization is not possible.
- The uniqueness of this study is that it was conducted at a high level of basketball during the competitive season, thus the findings of these studies are ecologically valid, with the methods being easily applied by coaches for programming to improve the physical performance of basketball players during the tapering phase before the post-season.
|
Basketball is a sport characterized by large number of high-intensity tasks such as jumping, sprinting, accelerating, decelerating, and changes of direction (CoD) (Stojanović et al., 2018). Previous studies reported that professional basketball players perform more than 300 CoD maneuvers, 50 accelerations and 50 jumps during a basketball game, indicating these movements as determinants of basketball performance (Svilar and Jukić, 2018). Therefore, evaluation of these tasks is an indispensable component of basketball training as it provides coaches and athletes with information about individual’s neuromuscular function and provides insight into an athlete’s weaknesses and strengths, based on which the training plan can then be customized (Morrison et al., 2022). Studies showed distinction between basketball players of different competitive levels, where better players can sustain high-intensity intermittent efforts for a longer duration and have better strength and power capabilities (Ferioli et al., 2020a; 2020b; Yáñez-García et al., 2022). Given the importance of strength and power qualities for basketball players (Dos’Santos et al., 2021; Riggs and Sheppard, 2009; Spiteri et al., 2014), an analytical approach to biomechanical testing can provide detailed insight into the athlete’s neuromuscular capacity, providing the opportunity for training optimization. Thus, it seems important that a strength and power-based program, individualized to the needs of the player, is implemented to maximize basketball performance. Strength and power capabilities can be represented on the force-time continuum. To do this and get a better insight into an athlete’s muscular mechanical capabilities we can assess the ability to express force under different loading conditions (e.g., high load and low load or only body weight). The magnitude of the difference in peak force between these two conditions can reflect adaptations of the neuromuscular system to the training process. To assess the ability to use force capacity to the greatest extent, it is logical to use a metric that combines force expression under different loadings (Comfort et al., 2018a; McMahon et al., 2017; Thomas et al., 2017). In particular, the dynamic strength index (DSI) has been recently suggested as a promising method for obtaining insights into an athlete’s strength and power qualities, providing a more comprehensive understanding of an individual’s capabilities and deficits (Bishop et al., 2021b; Comfort et al., 2018b; Suchomel et al., 2020). The DSI is calculated as the ratio between force production during ballistic (e.g., vertical jumps) and isometric/quasi-isometric tasks (e.g., isometric mid-thigh pull (IMTP)) (Comfort et al., 2018a; Sheppard et al., 2011; Young et al., 2014). In other words, the DSI reflects an athlete’s dynamic force production capacity relative to their maximal isometric force capacity. DSI was introduced as a metric that reflects individual training needs (Sheppard et al., 2011; McMahon et al., 2017). Low values of DSI indicate that the athlete should focus on low-load power training to generate higher forces during high-velocity conditions. In contrast, a high DSI suggests that the athlete should focus on maximal strength training to improve their capacity for maximal force production (Sheppard et al., 2011). Additionally, when using the DSI ratio, it is important to consider the relative effect of each component (force component and ballistic component), as increasing both components simultaneously would result in minimal changes in DSI but would likely have a positive impact on performance. It is well-documented that a short-term intervention (5-12 weeks), with a strength or ballistic training program in young males, significantly improves physical performance (Asadi, 2013; Barrera-Domínguez et al., 2023; Brini et al., 2022; Cormie et al., 2007; Ujaković and Šarabon, 2023; Wilson et al., 1993). Furthermore, literature suggests that a generalized strength or ballistic training program can improve the physical performance of basketball players to some extent (Cherni et al., 2021; Yáñez-García et al., 2022; Zhang et al., 2023). Previous intervention studies mostly used a “one-size-fits-all” strategy (Cherni et al., 2021; Yáñez-García et al., 2022) irrespective of players’ individual needs in terms of development specific physical capabilities. A limitation with this type of training intervention is that it does not consider the fluctuations in strength, power, biological variability, and fatigue during a long competitive basketball season and can thus be ineffective when training to improve or maintain an athlete’s physical performance (Cherni et al., 2021). On the other hand, literature indicates that individualized (in contrast to generalised) resistance training program is more effective for improving physical performance of basketball players (Barrera-Domínguez et al., 2023; Cherni et al., 2021). Moreover, if individualized, training interventions consisting of three exercises per session performed two times per week can be effective for improving physical performance of basketball players (Barrera-Domínguez et al., 2023). Based on existing literature, DSI has the potential to serve as a valuable metric for guiding the individualization of training processes aimed at enhancing athletic performance. Despite this potential, there have been no interventional studies investigating the efficacy of a program tailored to an individual’s DSI. Consequently, direct evidence supporting the utility of DSI for improving training individualization remains absent. The aim of this randomized controlled trial was to determine the effectiveness of the DSI to optimize training programs to improve physical performance proxies (sprinting, jumping and CoD) in basketball players. Based on this, the primary objective of the study was to examine the effect of an in-season individualized training program based on DSI results on the athletic performance (jumping, sprinting, and CoD) of professional basketball players in comparison to a standard (non-individualized) training program. In addition, we examined changes in DSI values based on different training types (i.e., strength, ballistic, control). Based on the main objective of this study we hypothesized that the intervention group (IG) would improve physical performance to a greater extent than the control group (CG). We also assume that DSI values would change accordingly to type of the training, resulting in an increase in DSI values in ballistic group, a decrease in DSI values in the strength group and will not change in the CG.
Study designWe used a parallel-group, randomized-controlled trial study design. The trial was registered within ClinicalTrails.gov database (record number: NCT06094075). Participants were randomly allocated to either the IG or the CG by drawing cards from opaque sealed envelopes. After the randomization to allocate participants into the IG and CG, participants in the IG were allocated into two groups based on DSI values. A 2:1 ratio was employed between the IG and CG to ensure a sufficient number of participants in the IG, facilitating subgroup analyses (based upon further allocation of the participants in the IG to either strength or ballistic training). Measurements and intervention occurred during the seven week in-season period, right before play-off period. Two resistance exercise sessions per week were performed in both groups, with participants in the IG performing the exercises individually tailored to their DSI values, and CG performing a non-individualized program. Pre- and post-intervention assessment included independent measures of athletic performance. Included a 20-m sprint, 505 CoD test, countermovement jump (CMJ) and IMTP.
ParticipantsWe used G*Power 3.1 software (Heinrich Heine University, Düsseldorf, Germany) for calculating sample size for a within-between analysis of variance (ANOVA) (effect size (f) = 0.26; α error = 0.05, and power = 0.80). The f-effect size was converted from eta2 of 0.06, which is the low bound for the moderate effect. This computation recommended a sample size greater than 34 participants. Due to potential dropouts, we recruited 43 highly trained basketball players (age = 19.4 ± 2.9 years; height = 1.97 ± 0.08 cm, weight = 89.1 ± 9.5 kg) to participate in the study. Participants were from three different teams, all competing at the same level. Nevertheless, all participants had five basketball training sessions, two games and two resistance training sessions per week during the study period. The randomization was performed in each team separately with the same procedure by drawing cards from opaque sealed envelopes. A CONSORT flow diagram of the progress of the participants through the phases of the trial is shown in Figure 1. All participants are part of high-level basketball teams, participating at national and international level with > five years of structured basketball training. Participants were eligible to take part in the study if they 1) had > two years of strength training experience and 2) had been injury-free with normal participation in all basketball activities for past six months. None of the participants took any medicine that would in any way influence the testing results or training procedure. Moreover, participants were asked to continue with their regular diet without any changes during the study procedures. The protocol was conducted in accordance with the latest revision of the Declaration of Helsinki. The experimental procedures were reviewed and approved by the Medical Ethics Committee (approval no. 0120-99/2018/5).
Intervention and assessmentThroughout the intervention period, all participants engaged in an average of 10 hours of training per week, which comprised five basketball sessions and two resistance training sessions which presents the typical weekly schedule for high-level basketball players during the competition period (Ujaković and Šarabon, 2023). In addition, they played two games weekly. Pre-test was performed one week before first resistance training session and post-test was performed one week after the training sessions had finished. Players performed both testing sessions at the same time of the day in the afternoon (± one hour) to avoid the influence of the circadian rhythm. The order of the subjects was randomised for each testing session. The measurements were done in basketball hall with air conditioning, with air conditioning, with the temperature kept between 20 and 22° and humidity between 40 and 60%. Moreover, testing was done the next day after the off day, to prevent fatigue that would influence testing results. Testing was performed at the same location and same training gym at pre- and post-intervention, with the field tests being performed on the basketball court, while jumps and strength test were performed in the neighbour room (fitness room), where is the solid floor to avoid signal interruptions when gathering data with force plates. All assessments were conducted by investigators who held at least a master’s degree in sport science and had ample practical experience with conducting the tests in elite-level athletes. Each player performed a 20-m sprint, 505 CoD test, CMJ and IMTP. Based on CMJ peak force and IMTP peak force data we calculated DSI (Thomas et al., 2017). Based on the mean DSI value (0.89), the participants in the IG were divided into two groups - strength group (DSI > 0.9; n = 16), ballistic group (DSI ≤ 0.9; n = 11). DSI benchmark to allocate participants into strength or ballistic group was consistent with available literature regarding DSI in basketball players, indicating mean values of 0.86-0.90 (Thomas et al., 2017). The resistance training program consisted of two sessions per week for five weeks (10 sessions all together) and are shown in Table 1. The sessions were separated by minimum of 48 hours (Cherni et al., 2021). The strength training group consisted of mostly exercise with high loads, whereas the ballistic training group performed dynamic/explosive exercises with low loads or bodyweight exercises (Barrera-Domínguez et al., 2023). Other training variables were the same for both groups, consisting of three exercises per training and total six exercises per week (Barrera-Domínguez et al., 2023), with four of the exercises being bilateral, one unilateral and one quasi-unilateral (Bulgarian split squat and scissor jumps). Five exercises were multi-joint tasks, while one single-joint exercise was chosen to specifically target ankle joints. The CG performed regular resistance training consisting of combination of similar (basic) strength and ballistic exercises as IG. Resistance training of the CG included three to four lower body exercises per session with two of them being strength exercises (e.g. squats, Bulgarian split squat, trap bar deadlift or calf raises) and one or two plyometric exercises (e.g. depth jumps, pogo jumps or weighted jumps). For all exercises three sets were done with similar rep ranges and intensities as in the IG. During the experiment all players performed their regular prevention program and upper body program determined by their strength and conditioning coach. The resistance training sessions were supervised by strength and conditioning coaches which were previously trained by research team to ensure proper execution of the programs (Coleman et al. 2023). The intensity of the strength exercises was controlled using % of RM (which was regularly tested by strength and conditioning coaches to determine exercise intensity) and self-reported reps in reserve (RIR) with rep ranges that correspond to determined intensity (Helms et al. 2018), with which they were already familiarized as this is regularly used method by their strength and conditioning coaches to determine exercise intensity. Assessment was performed one week before the first training session and the following week after the training sessions had finished. First, the participants performed a standardized warm-up, consisting of five min of jogging, dynamic stretching, and body-weight strength exercises (squats, all-direction lunges, and planks), followed by three warm up repetitions of CMJs and three gradual accelerations to full sprint speed. The warm-up was followed by 20-m sprint test, 505 CoD test, CMJ and IMTP. There was a 10 min break after the field tests, before jumping and IMTP assessment. Athletes have been performing all tests as a regular testing routine before, therefore, no familiarization was needed.
Linear sprintThe linear sprint was performed over a distance of 20-m, with timing gates (Brower Timing Systems, Draper, UT, United States) set up at 5-m intervals (i.e. 5, 10, and 15-m) (Pleša et al., 2023). Participants began each sprint 0.3 m behind the starting line to prevent early triggering. The start was from a standing position, and participants were free to choose their front leg, which was kept constant throughout all repetitions. They were instructed to sprint from the start line as fast as possible through both sets of timing gates. A total of three runs were undertaken, each separated by a two min rest, with the best repetition being taken for further analysis.
Change of directionCoD was determined with th e 505-test, employing the same timing gate device (Brower Timing Systems, Draper, UT, United States). Participants were instructed to sprint to a line 15 m from the start line (the time gates were positioned 10 m from the start line), plant their left or right foot on the line, turn 180°, and sprint 5 m back through the timing gates (Nimphius et al., 2016). To prevent early triggering, participants started 0.3 m behind the starting line (as in the sprint test). This sequence was repeated thrice for each leg, alternating between the left and right, with a two min rest interval between each repetition, and the best repetition being taken for further analysis. Furthermore, as proposed by (Nimphius et al., 2016), the CoD deficit was computed to offer a more distinct measure of CoD performance. This deficit was determined by subtracting the 10-m linear sprint time from the 505-test time. Essentially, the CoD deficit represents the added time a player requires to complete a CoD task in comparison to a straightforward acceleration over an identical distance (Nimphius et al., 2016). CoD deficit was calculated from the best CoD and sprint repetitions with that value being taken for further analysis.
Vertical jumpsGround reaction force data during jumps and IMTP were sampled with a piezoelectric force platform (Type 9229A, Kistler Instruments, Winterthur, Switzerland) at 1000 Hz and stored within the MARS Software (Kistler, Winterthur, Switzerland), which enables immediate and reliable calculation of the biomechanical jump variables (Hébert-Losier and Beaven, 2014). Following three familiarization repetitions at submaximal effort, three repetitions of CMJ were performed with one min rest between. Participants were instructed to perform jump as fast and as high possible, with self-selected countermovement depth (Ebben and Petushek, 2010). Throughout the duration of each jump, hands were to be maintained on the hips. Time to take off was determined as the time between the countermovement initiation (defined as the decrease in force signal larger than three standard deviations of the baseline signal) and the take-off (defined as the first instant of force < 10 N). The impulse was then calculated by integrating the force-time curve during the take-off phase. Utilizing the impulse-momentum theorem, the take-off velocity was determined, and jump height was estimated from the take-off velocity based on the kinematic flight equations. Jump height, jump time and reactive strength index (RSI) were considered as indicators of physical performance, while peak force was taken to calculate DSI. RSI was calculated by dividing the jump height with the time to take off (Ebben and Petushek, 2010).
Isometric mid-thigh pull and dynamic strength indexThe IMTP tests were performed on the same force platform as the jumping assessments (Type 9229A, Kistler Instruments, Winterthur, Switzerland). Position was determined as the strongest position to pull the bar chosen by players, with knees at ~40° (Comfort et al., 2018b). The height of the bar (i.e., the distance from force plate to the bar) was measured and was kept the same during pre- and post-intervention measurements for each player. Participants had to exert maximal force against a bar that was firmly connected to the ground so that it could only be lifted to a pre-determined height (Figure 2). After two warm-up repetitions at ~50% and ~75% of maximal effort, three repetitions with maximal exertion were performed with a two min rest between repetitions. The average value of the three repetitions was taken for further analysis. In IMTP test, the peak force was taken as the largest mean force in one second intervals. Subsequently, DSI variables were calculated from average value of peak force obtained in CMJ, and average value of peak force obtained during IMTP test as follows:

The primary variables included performance proxies (sprint times, 505 times, CoD deficit and CMJ parameters). The secondary analysis also considered the DSI and its constituent variables (CMJ peak force and IMTP peak force).
Statistical analysisThe data are presented as means ± standard deviations. The normality of the data distribution for all variables was verified with the Shapiro-Wilk test. Trial-by-trial reliability was determined with a two-way random intraclass correlation coefficient (ICC) with absolute agreement and 95% confidence intervals (CI) and coefficient of variation (CV). ICC was interpreted as following: > 0.9 (excellent), 0.9-0.75 (good), 0.75-0.50 (moderate), and < 0.5 (poor) (Koo and Li, 2016). Absolute reliability was assessed by calculating the coefficient of variation (CV) according to Hopkins (2000) and interpreted as poor (CV >10%), moderate (CV = 5-10%), or good (CV <5%) (Banyard et al., 2017). Univariate analysis of covariance (ANCOVA) was used to determine the difference between groups in post-intervention values while using pre-intervention values as covariate, with effect sizes (eta squared; η2) interpreted as small (~0.01), moderate (~0.06), or large (~0.14) (Cohen, 2013). Furthermore, post-hoc testing was done with paired t-tests with Bonferroni correction to measure statistical differences between pre-intervention and post-intervention results within groups. Hedges g with 95% confidence intervals was used to determine within-group effect size between pre- and post-intervention results and was interpreted as following: trivial (< 0.2), small (0.2-0.5), medium (0.5-0.8) and large (> 0.8) (Lakens, 2013). The threshold for statistical significance was set at α < 0.05 and all analyses were carried out in statistical software Statistical Product and Service Solutions (SPSS) (version 25.0, IBM, USA).
All participants completed 10/10 training sessions throughout five weeks. At baseline, sixteen participants were directed towards strength training (mean DSI = 0.98; range = 0.92-1.12 and eleven towards ballistic training (DSI = 0.82; range = 0.71-0.90). Sixteen participants were allocated to the CG (DSI = 0.86; range = 0.70-1.12) and continued with their general training program. Comparison analysis in IG included participants of both ballistic and strength groups together. Table 2 shows absolute and relative trial-by-trial reliability for all tests included in this study at pre-intervention and post-intervention assessments. All data at pre-intervention and post-intervention time shows excellent relative reliability with ICC ≥ 0.94 and good to moderate absolute reliability with CV ≤ 5.72 %. Table 3 shows mean and standard deviation (SD) for performance variables for each group at pre-intervention and post-intervention time points and changes that occurred from pre- to post-intervention. ANCOVA indicated a statistically significant shorter sprint times (p = 0.002-0.010) and 505-test times (p = 0.008-0.009) during post-intervention assessments for the IG compared to the CG, with large effect size (η2 = 0.116-0.209). There were no statistically significant differences for CMJ variables and CoD deficit (p = 0.551-0.837). Post-hoc analysis within groups showed statistically significant improvements (p = 0.027, g = 0.46) in 5-m sprint performance in IG, while performance significantly decreased in CG in 5, 10 and 20-m sprint distance (p = 0.004-0.015 g = -0.42 to -0.62). Furthermore, the IG significantly improved performance in 505 test (p = 0.006-0.025, g = 0.46-0.47), while changes in 505 performances were not significant in the CG (p = 0.085-0.126), with the trend showing decrease in performance. CoD deficit values tended to decrease in both groups, but the difference was not statistically significant (p = 0.184-0.480). Table 4 shows mean and SD for basic variables for each group at both time points. ANCOVA indicated a statistically significant difference between groups at IMTP relative force (p = 0.039, η2 = 0.108), during post-intervention assessments, while post-hoc testing showed significant differences between pre- and post-intervention only in IMTP peak force in IG (p = 0.025). Although not significant (p = 0.186-0.177) CG slightly increased force production in CMJ and decreased IMTP force, resulting in significant increase in DSI values (p = 0.049). On the other hand, IG significantly increase IMTP force (p = 0.025), with no change in CMJ force production (p = 0.939), trending towards decrease in DSI values. Additional subgroup analysis was done which considered the control, ballistic, and strength groups independently to discern the differential effects of the respective training protocols. Again, ANCOVA indicated statistically significant differences at all sprint split times (p = 0.004-0.018; η2 = 0.187-0.248) and 505 variables (p = 0.029-0.028, η2 = 0.167) and IMTP relative force (p = 0.032, η2 = 0.169) during post-intervention assessments. Post-hoc tests showed significant differences between pre- and post- intervention results in CMJ height (p = 0.039), 5-m (p = 0.008) and 10-m (p = 0.035) sprint and 505 test (p = 0.038) on left side in strength group, while no significant differences were observed in ballistic group. Both groups tended towards an increase in CMJ, sprint and 505 performances. Furthermore, although no significant differences were observed in DSI in both groups (p = 0.301-0.424), DSI values decreased in the strength group and did not change in the ballistic group. Results with means and SD for all variables are presented in Table 5.
The aim of the present study was to investigate the effects of a five-week individualized training based on the dynamic strength index on sprinting, jumping, and CoD performance in highly trained basketball players. The main finding of the study is that the IG exhibited significant improvements in jumping, the 5m sprint, and CoD performance in the 505 test, while the CG significantly decreased sprint performance at all distances measured (5-20 m). Furthermore, our results show a significant increase in DSI values in the CG, which is underpinned by a tendency towards a decrease in IMTP force and an increase in CMJ force, while the IG tended to decrease DSI values, underpinned by a significant increase in IMTP force. Although both intervention subgroups tended towards improvement in performance proxies, statistically significant improvements were found only in the strength group. Furthermore, the strength group decreased DSI values, while in the ballistic group, DSI values did not change from pre- to post-intervention. Results of this study showed that evaluating DSI values and programming training based on these values were effective for improving the physical performance of basketball players during a five-week training period. In particular, participants with very high DSI values largely benefited from individualized training with high loads. Our results indicate that DSI may be sensitive to detect specific neuromuscular deficits, based on which training loads might be adjusted to improve physical performance. While solid support for using DSI in practice is lacking in the literature, force-velocity profiling (FVP) is well established in literature to be an effective tool to guide training related decisions (Jiménez-Reyes et al. 2017; Ramirez-Campillo et. al., 2022). FVP is obtained through testing athlete’s qualities across different loading conditions (Jiménez-Reyes et al. 2017), which allows the identification of the mechanical capabilities of musculoskeletal system to produce force, power and velocity (Jaric, 2015; Samozino et al., 2016) FVP slope navigates individuals training (Ramirez-Campillo et. al., 2022), with athletes having steeper FVP profile slopes (i.e., having relatively high F0 and low V0) being better at generating high forces at low velocities thus being directed towards velocity oriented training and vice versa (Jiménez-Reyes et al., 2017). Previous literature has attempted to compare FVP in vertical jump and DSI (Pleša et al., 2023). The study reported that individuals with higher DSI scores had a more velocity dominant profile (higher V0), indicating that they are relatively better in producing force at high velocities (Jiménez-Reyes et al., 2017). Conversely, individuals with lower DSI scores had a steeper FV slope (i.e., can express a lower percentage of their maximal isometric force ability at higher velocities), which implies that they need to put more emphasis on ballistic training (Pleša et al., 2023). Based on these results it seems that DSI training theory goes in line with the FV relationship training theory. Similar to DSI-based training in our study, individually tailored training based on FVP has been shown to be effective for improving physical performance of basketball players (Barrera-Domínguez et al., 2023). Training based on FVP individualization showed significant changes in IG in vertical jump and sprint after four weeks, while changes in CoD were observed only after eight weeks (Barrera-Domínguez et al., 2023). Our study observed significant improvements in jump, sprint, and CoD performance after five weeks. Unlike the previous off-season study with three basketball practices and two resistance training sessions per week, our study occurred during the basketball season, involving five practices, two resistance sessions, and two games weekly. Furthermore, we observed significant performance decrements in CG for sprint performance with the tendency towards performance decrements also in CoD tests. The deterioration in performance on tests from pre- to post-intervention in the CG is consistent with previous studies, which highlights that accumulated fatigue during a basketball season can adversely affect neuromuscular performance (Stojanović, et al., 2018). Notably, the IG displayed a significant increase in IMTP force, while the CG showed a trend toward a reduction in IMTP force. This difference in IMTP force change between the two groups could potentially explain the variations in sprint and CoD performance, given that strength is an important determinant of and CoD capabilities (Dos’Santos et al. 2021; Castillo-Rodríguez et al., 2012). Performance decrement may be due to the accumulated fatigue from the overload of the training and gameplay (Stojanović, et al., 2018; Bishop et al., 2021a; Petway et al. 2020; Ujaković and Šarabon, 2023). It seems that individually tailored resistance exercise performed twice per week is more effective for maintaining (in case of CMJ) or even improving (in case of sprint and CoD) physical performance within the competitive basketball seasons. Based on our results, it seems that individualized resistance training may be crucial to sustain or improve the physical performance of basketball players during this period. Nevertheless, it would be interesting for the future research to follow seasonal fluctuations in DSI and its components to get more information about mechanisms behind the performance deterioration. The rationale for selecting a DSI value of 0.9 as a benchmark for participant allocation was based on the lack of established benchmarks in existing literature to guide practitioners in making training-related decisions. It is crucial to note that appropriate benchmarks are highly dependent on the specific task at hand. For instance, previous research conducted by Thomas et al. (2017) has illustrated variations in DSI values across different team sports, demonstrating that basketball players tend to have higher DSI values in comparison to their counterparts in cricket and soccer. Furthermore, literature shows that DSI values may vary between testing approaches (squat jump vs. CMJ for ballistic task, squat vs. IMTP for the isometric task) (Pleša et al., 2023; Thomas et al. 2017). Preliminary evidence suggests that the DSI values are highly variable among sports, testing approaches and sex, thus, it is not feasible to follow one-size fits-all benchmarks. To the author’s knowledge one study attempted to set DSI benchmark to guide training related decisions (Sheppard et al. 2011). Authors reported that a DSI < 0.6 might be considered low, suggesting ballistic training to generate higher forces during the ballistic task. Conversely, a high DSI (> 0.80) indicates a need for maximal strength training to enhance maximal force production. However, this was a pilot study with only five participants from various sports, ages, and sexes, differing in training volume (two to three sessions/week), duration (8-10 weeks), and programs. Available literature regarding DSI in basketball players, including our present study, has reported mean DSI values at ~0.86-0.90 (Thomas et al., 2017). High baseline DSI values in basketball players might be due to the nature of the sport, consisting of many explosive movements such as sprints, jumps, and CoDs. If we followed the benchmarks put forward by Sheppard et. al. 2011, all players in our study would be directed towards strength training or combination of strength and power training. Similarly, in a study using FVP for navigating resistance training, most (but not all) basketball players are more velocity oriented and/or force deficient (Ramirez-Campillo et. al., 2022). While the abovementioned study reported significant improvements in IG, they did not report about separate analysis regarding the type of the training (i.e., strength training or velocity emphasized training). Similarly, to their results, IG in our study improved sprint and CoD performance, while additional analysis showed that only strength group significantly improved physical performance, while improvements in ballistic group were not significant. It may be that strength training is preferable during the basketball season for improving physical performance, as basketball players are largely velocity-dominant athletes. Hence, the benchmark for allocating participants into strength or ballistic group was possibly set too high in the present study, and it could be that a larger portion of participants would benefit more from strength training than from ballistic training. Therefore, more studies are needed to determine exact DSI benchmark to navigate resistance training in basketball players during the basketball season. Nevertheless, results of this study indicate that short strength training intervention may be useful strategy for maintenance of physical performance during the periods of high demand and fatigue in competitive basketball as well as useful method in tapering phase when preparing for the final part of the season. To our knowledge, there is only one study that has investigated the effects of resistance training on DSI itself (Comfort et al., 2018a). The authors reported a decrease in DSI scores in a “high” DSI group after four weeks of mixed strength and power training, while no changes were observed in the “low” DSI group. Similar to our results, the changes in DSI were largely underpinned by an increase in IMTP peak force, with minimal or no change in CMJ peak force in the strength group, while the CG significantly increased DSI values, underpinned by an increase in CMJ peak force and a decrease in IMTP peak force. Interestingly, the ballistic group did not change DSI values. These results indicate that DSI should always be interpreted in conjunction with both underlying peak force variables. It would be interesting to see future research include velocity-based training intervention to get insight into how certain velocity thresholds and velocity drops influence DSI values. The results of this study should be interpreted with caution. Although the IG showed superior results compared to the CG, additional analysis revealed that significant improvements in physical performance were observed only in the strength group. Although the ballistic group tended toward improvements in physical performance, no significant changes were observed. Additional studies are needed to determine the precise benchmarks for navigating training based on DSI values. Furthermore, more studies are necessary to ascertain the effectiveness of different resistance training methods during the basketball season on the physical performance of basketball players. Moreover, this study was conducted in the period right before the post-season with a very homogeneous sample, so the results cannot be generalized to performance metrics and athletes from other sports. Finally, we were not able to control the volume of basketball games, as the decisions about who plays and how much they play are entirely up to the head coaches; thus, some discrepancies may be present between individuals. Nevertheless, while it is extremely difficult to conduct this type of research at a high level, due to limited time and opportunities during the basketball season, we find that controlling for resistance training and randomization when creating the CG and IG is sufficient.
Based on the results of this study, it can be suggested that evaluating DSI values and programming training based on these values can be an efficient way to improve performance during the tapering phase before the post-season. In conclusion, this study shows that three sets of three exercises, performed twice a week and selected based on the DSI ratio, could be sufficient to improve (or prevent a decrease in) the physical performance of basketball players during the basketball season. Furthermore, this type of intervention is easily implemented into a system of physical preparation, which makes it suitable to be executed during the basketball season.
ACKNOWLEDGEMENTS |
The study was supported by the Slovenian Research Agency through the research program KINSPO - Kinesiology for the effectiveness and prevention of musculoskeletal injuries in sports (P5-0443). The authors declare no potential or actual conflicts of interest. The datasets generated and analyzed during the current study are not publicly available, but are available from the corresponding author who was an organizer of the study. The experiments comply with the current laws of the country where they were performed. |
|
AUTHOR BIOGRAPHY |
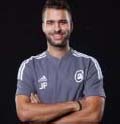 |
Jernej Pleša |
Employment: Basketball club Cedevita Olimpija |
Degree: MSc |
Research interests: Strength and conditioning, sports performance. |
E-mail: jernej.plesa@hotmail.com |
|
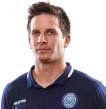 |
Filip Ujaković |
Employment: Basketball club Cedevita Olimpija |
Degree: PhD |
Research interests: Strength and conditioning. |
E-mail: fujakovic@gmail.com |
|
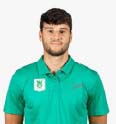 |
Ažbe Ribič |
Employment: Soccer club Olimpija Ljubljana |
Degree: MSc |
Research interests: Strength and conditioning, sports performance. |
E-mail: azberibic98@gmail.com |
|
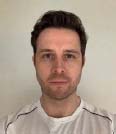 |
Chris Bishop |
Employment: London Sport Institute, Middlesex University |
Degree: PhD |
Research interests: Strength and conditioning, inter-limb asymmetries. |
E-mail: C.Bishop@mdx.ac.uk |
|
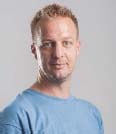 |
Nejc Šarabon |
Employment: University of Primorska, Faculty of Health Sciences, Slovenia |
Degree: PhD |
Research interests: Strength and conditioning, Motor control, Physical therapy |
E-mail: nejc.sarabon@fvz.upr.si |
|
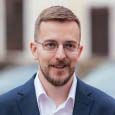 |
Žiga Kozinc |
Employment: University of Primorska, Faculty of Health Sciences, Slovenia |
Degree: PhD |
Research interests: Strength and conditioning, Motor control, Physical therapy |
E-mail: ziga.kozinc@fvz.upr.si |
|
|
|
REFERENCES |
 Asadi A. (2013) Effects of In-season Short-term Plyometric Training on Jumping and Agility Performance of Basketball Players. Sport Sciences for Health 9, 133-137. Crossref
|
 Banyard H. G., Nosaka K., Vernon A. D., Haff G. G. (2018) The reliability of individualized load–velocity profiles. International journal of sports physiology and performance 13, 763-769. Crossref
|
 Barrera-Domínguez J.F., Almagro J.B., de Villarreal E.S., Molina-López J. (2023) Effect of Individualised Strength and Plyometric Training on the Physical Performance of Basketball Players. European Journal of Sport Science 23, 2379-2388. Crossref
|
 Bishop C., Read P., Lake J., Loturco I., Turner A. (2021b) A Novel Approach for Athlete Profiling: The Unilateral Dynamic Strength Index. Journal of Strength and Conditioning Research 35, 1023-1029. Crossref
|
 Bishop C., Weldon A., Hughes J., Brazier J., Loturco I., Turner A., Read P. (2021a) Seasonal Variation of Physical Performance and Inter-limb Asymmetry in Professional Cricket Athletes. Journal of Strength and Conditioning Research 35, 941-948. Crossref
|
 Brini S., Boullosa D., Calleja-González J., Van Den Hoek D.J., Nobari H., Clemente F.M. (2022) Impact of Combined Versus Single-Mode Training Programs Based on Drop Jump and Specific Multidirectional Repeated Sprint on Bio-Motor Ability Adaptations: A Parallel Study Design in Professional Basketball Players. BMC Sports Science, Medicine, and Rehabilitation 14, 160. Crossref
|
 Castillo-Rodríguez A., Fernández-García J.C., Chinchilla-Minguet J.L., Carnero E.Á. (2012) Relationship Between Muscular Strength and Sprints with Changes of Direction. Journal of Strength and Conditioning Research 26, 725-732. Crossref
|
 Cherni Y., Hammami M., Jelid M. C., Aloui G., Suzuki K., Shephard R.J., Chelly M.S. (2021) Neuromuscular Adaptations and Enhancement of Physical Performance in Female Basketball Players After 8 Weeks of Plyometric Training. Frontiers in Physiology 11, 588787. Crossref
|
 Cohen, J. (2013) Statistical Power Analysis for the Behavioral Sciences.
New York: Routledge. Crossref
|
 Coleman M., Burke R., Benavente C., Piñero A., Augustin F., Maldonado J., Fisher J.P., Oberlin D., Vigotsky A.D., Schoenfeld B.J. (2023) Supervision During Resistance Training Positively Influences Muscular Adaptations in Resistance-Trained Individuals. Journal of Sports Sciences 41, 1207-1217. Crossref
|
 Comfort P., Thomas C., Dos’Santos T., Jones P.A., Suchomel T.J., McMahon J. J. (2018b) Comparison of Methods of Calculating Dynamic Strength Index. International Journal of Sports Physiology and Performance 13, 320-325. Crossref
|
 Comfort P., Thomas C., Dos’Santos T., Suchomel T.J., Jones P.A., McMahon J. J. (2018a) Changes in Dynamic Strength Index in Response to Strength Training. Sports (Basel, Switzerland) 6, 176. Crossref
|
 Cormie P., McCaulley G.O., McBride J.M. (2007) Power Versus Strength-Power Jump Squat Training: Influence on the Load-Power Relationship. Medicine and Science in Sports and Exercise 39, 996-1003. Crossref
|
 Dos’Santos T., Thomas C., McBurnie A., Comfort P., Jones P.A. (2021) Biomechanical Determinants of Performance and Injury Risk During Cutting: A Performance-Injury Conflict?. Sport Medicine 51, 1983-1998. Crossref
|
 Ebben W. P., Petushek E. J. (2010) Using the reactive strength index modified to evaluate plyometric performance. The Journal of Strength & Conditioning Research 24, 1983-1987. Crossref
|
 Ferioli D., Bosio A., Zois J., La Torre A., Rampinini E. (2020a) Seasonal Changes in Physical Capacities of Basketball Players According to Competitive Levels and Individual Responses. Plos One 15, e0230558. Crossref
|
 Ferioli D., Schelling X., Bosio A., La Torre A., Rucco D., Rampinini E. (2020b) Match Activities in Basketball Games: Comparison Between Different Competitive Levels. Journal of Strength and Conditioning Research 34, 172-182. Crossref
|
 Hébert-Losier K., Beaven C.M. (2014) The MARS for Squat, Countermovement, and Standing Long Jump Performance Analyses: Are Measures Reproducible?. Journal of Strength and Conditioning Research 28, 1849-1857. Crossref
|
 Helms E.R., Cross M.R., Brown S.R., Storey A., Cronin J., Zourdos M.C. (2018) Rating of Perceived Exertion as a Method of Volume Autoregulation Within a Periodized Program. Journal of Strength and Conditioning Research 32, 1627-1636. Crossref
|
 Jaric S. (2015) Force-velocity Relationship of Muscles Performing Multi-joint Maximum Performance Tasks. International Journal of Sports Medicine 36, 699-704. Crossref
|
 Jiménez-Reyes P., Samozino P., Brughelli M., Morin J.B. (2017) Effectiveness of an Individualized Training Based on Force-Velocity Profiling During Jumping. Frontiers of Physiology 7, 677. Crossref
|
 Koo T.K., Li M.Y. (2016) Guideline of Selecting and Reporting Intraclass Correlation Coefficients for Reliability Research. Journal of Chiropractic Medicine 15, 155-163. Crossref
|
 Lakens D. (2013) Calculating and Reporting Effect Sizes to Facilitate Cumulative Science: A Practical Primer for T-tests and ANOVAs. Frontiers in Psychology 4, 863. Crossref
|
 McMahon J.J., Jones P.A., Dos’santos T., Comfort P. (2017) Influence of Dynamic Strength Index on Countermovement Jump Force-, Power- Velocity-, and Displacement-Time Curves. Sports 5. Crossref
|
 Morrison M., Martin D. T., Talpey S., Scanlan A.T., Delaney J., Halson S.L., Weakley J. (2022) A Systematic Review on Fitness Testing in Adult Male Basketball Players: Tests Adopted, Characteristics Reported and Recommendations for Practice. Sports Medicine 52, 1491-1532. Crossref
|
 Nimphius S., Callaghan S. J., Spiteri T., Lockie R. G. (2016) Change of direction deficit: A more isolated measure of change of direction performance than total 505 time. The Journal of Strength & Conditioning Research 30, 3024-3032. Crossref
|
 Petway A.J., Freitas T.T., Calleja-González J., Torres-Ronda L., Alcaraz P.E. (2020) Seasonal Variations in Game Activity Profiles and Players’ Neuromuscular Performance in Collegiate Division I Basketball: Non-conference vs. Conference Tournament. Frontiers in Sports and Active Living 2, 592705. Crossref
|
 Pleša J., Kozinc Ž., Bishop C., Šarabon N. (2023) Association Between Dynamic Strength Index Derived from Isometric Squat and Squat Jump or Countermovement Jump and Force-Velocity Profile. Measurement in Physical Education and Exercise Science 28, 57-65. Crossref
|
 Ramirez-Campillo R., García-Hermoso A., Moran J., Chaabene H., Negra Y., Scanlan A.T. (2022) The Effects of Plyometric Jump Training on Physical Fitness Attributes in Basketball Players: A Meta-Analysis. Journal of Sport and Health Science 11, 656-670. Crossref
|
 Riggs M.P., Sheppard J.M. (2009) The Relative importance of Strength and Power Qualities to Vertical Jump Height of Elite Beach Volleyball Players During the Counter-Movement and Squat Jump. Journal Human Sport and Exercise 4, 221-236. Crossref
|
 Samozino P., Rabita G., Dorel S., Slawinski J., Peyrot N., Saez de Villarreal E., Morin J.B. (2016) A Simple Method for Measuring Power, Force, Velocity Properties, and Mechanical Effectiveness in Sprint Running. Scandinavian Journal of Medicine and Science in Sports 26, 648-658. Crossref
|
 Sheppard J.M., Chapman D., Taylor K.L. (2011) An Evaluation of a Strength Qualities Assessment Method for the Lower Body. The Journal of Australian Strength and Conditioning 19, 4-10.
|
 Spiteri T., Nimphius S., Hart N.H., Specos C., Sheppard J.M., Newton R.U. (2014) Contribution of Strength Characteristics to Change of Direction and Agility Performance in Female Basketball Athletes. Journal of Strength and Conditioning Research 28, 2415-2423. Crossref
|
 Stojanovi? E., Stojiljkovi? N., Scanlan A.T., Dalbo V. J., Berkelmans D.M., Milanovi? Z. (2018) The Activity Demands and Physiological Responses Encountered During Basketball Match-Play: A Systematic Review. Sports Medicine (Auckland, N.Z.) 48, 111-135. Crossref
|
 Suchomel T.J., Sole C.J., Bellon C.R., Stone M.H. (2020) Dynamic Strength Index: Relationships with Common Performance Variables and Contextualization of Training Recommendations. Journal of Human Kinetics 74, 59-70. Crossref
|
 Svilar L., Juki? I. (2018) Load Monitoring System in Top-Level Basketball Team. Kinesiology 50, 25-33. Crossref
|
 Thomas C., Dos’santos T., Jones P.A. (2017) A Comparison of Dynamic Strength Index Between Team-Sport Athletes. Sports 5. Crossref
|
 Ujakovi? F., Šarabon N. (2023) The Effects of Workload Difference Between Limbs in Plyometric and Strength Exercises in Reducing Asymmetry in Change of Direction Ability During Basketball Season. Journal of Strength and Conditioning Research 37, 2282-2288. Crossref
|
 Wilson G. J., Newton R. U., Murphy A. J., Humphries B. J. (1993) The Optimal Training Load for the Development of Dynamic Athletic Performance. Medicine and Science in Sports and Exercise 25, 1279-1286. Crossref
|
 Yáñez-García J.M., Rodríguez-Rosell D., Mora-Custodio R., González-Badillo J.J. (2022) Changes in Muscle Strength, Jump, and Sprint Performance in Young Elite Basketball Players: The Impact of Combined High-Speed Resistance Training and Plyometrics. Journal of Strength and Conditioning Research 36, 478-485. Crossref
|
 Young K.P., Haff G.G., Newton R.U., Sheppard J.M. (2014) Reliability of a Novel Testing Protocol to Assess Upper-Body Strength Qualities in Elite Athletes. International Jounral of Sports Physiolology and Performance 9, 871-875. Crossref
|
 Zhang M., Liang X., Huang W., Ding S., Li G., Zhang W., Li C., Zhou Y., Sun J., Li D. (2023) The Effects of Velocity-Based Versus Percentage-Based Resistance Training on Athletic Performances in Sport-Collegiate Female Basketball Players. Frontiers in Physiology 13, 992655. Crossref
|
|
|
|
|
|
|