|
|
|
ABSTRACT |
The aims of this investigation were to determine the evolution of selected turn variables during competitive backstroke races and to compare these kinematic variables between two different levels of swimmers. Sixteen national and regional level male swimmers participant in the 200 m backstroke event at the Spanish Swimming Championships in short course (25 m) were selected to analyze their turn performances. The individual distances method with two-dimensional Direct Linear Transformation (2D-DLT) algorithms was used to perform race analyses. National level swimmers presented a shorter “turn time”, a longer “distance in”, a faster “underwater velocity” and “normalized underwater velocity”, and a faster “stroking velocity” than regional level swimmers, whereas no significant differences were detected between levels for the “underwater distance”. National level swimmers maintained similar “turn times” over the event and increased “underwater velocity” and “normalized underwater velocity” in the last (seventh) turn segment, whereas regional level swimmers increased “turn time” in the last half of the race. For both national and regional level swimmers, turn “underwater distance” during the last three turns of the race was significantly shorter while no significant differences in distance into the wall occurred throughout the race. The skill level of the swimmers has an impact on the competitive backstroke turn segments. In a 200 m event, the underwater velocity should be maximized to maintain turn proficiency, whereas turn distance must be subordinated to the average velocity. |
Key words:
Swimming, biomechanics, motor skills, repeated measures
|
Key
Points
- The underwater turn velocity is as a critical variable related to the swimmers’ level of skill in a 200 m backstroke event.
- Best swimmers perform faster but no longer turn segments during a 200 m backstroke event.
- Best swimmers maintain their turn performance throughout the 200 m backstroke event by increasing the underwater velocity during the final part of the race.
- The turn distance out seems to be subordinated in order to maximize the average velocity during a 200 m backstroke event.
|
Performance in competitive swimming is determined by the time employed to complete the race distance. According to a deterministic model (Chow, 2011), the race distance is the sum of the start, the free swim and the turn segments. Therefore, performance can also be determined by the start, free swim and turn time. The main goal in the turn is to change the direction in the shortest time as possible to obtain the highest speed in the opposite direction (Tourny-Chollet et al., 2002). The turn segment is a major contributor to the race distance (Thayer and Hay, 1984) and could represent up to one third of the total race time in the 200 m breaststroke event (Blanksby et al., 1998). As race distance increases, from 50 to 1500 m, the turn time is even more important (Chow et al., 1984; Tourny-Chollet et al., 2002) as the underwater phase is repeated in every lap (Burkett et al., 2010). Coaches and swimmers should be aware that faster turns could compensate slower swimming phases and, therefore, they could have a crucial impact on swimming performance (Zamparo et al., 2012). In the backstroke turn, the swimmer rolls from a supine to a prone position and then performs a tumble turn (Blanksby et al., 2004). After the tumble turn, the swimmer must push forcefully from the pool wall and maintain a high velocity by kicking during the underwater phase (Zamparo et al., 2012). The kinematic analyses of the competitive turn have measured the time between two reference marks, usually at 7.5 m off the turning wall (Arellano et al., 1994; Shimadzu et al., 2008; Tourny-Chollet et al., 2002). However, some authors have addressed that backstroke swimmers often surpass those 7.5 m during the underwater phase of the turn (Blanksby et al., 2004; Kennedy et al., 1990). If the velocity during the underwater turn phase is faster than the stroking velocity, a gain of speed could occur when extending the underwater phase (Blanksby et al., 1998; Hubert et al., 2006; Tourny-Chollet et al., 2002). In fact, the distance of swimming resumption (“underwater distance”) has been related to the starting (Seifert et al., 2006; Vantorre et al., 2010b) and the breaststroke and butterfly turning (Blanksby et al., 1998; Tourny-Chollet et al., 2002) performances. When measuring the turn segment with fixed reference marks, it was observed that the best swimmers employed less time turning (Arellano et al., 1994; Kjendlie et al., 2006; Thomson et al., 2000). However, a stroking phase from the reference mark to the wall was included into the turn segment despite having been reported that the stroking and the turning skills are not directly correlated (Mason and Cossor, 2001). The higher skilled swimmers seemed to shorten the turning times by increasing head to wall distance at the beginning of the turn, by extending the legs (between 100° and 120°) at contact to reduce contact time (Araujo et al., 2010; Puel et al., 2012) and by kicking efficiently in the first meters after the turn (Zamparo et al., 2012). In a race situation, the best swimmers have also been reported to cope with fatigue and to pace themselves throughout the event (Chollet et al., 1997; Letzelter and Freitag, 1983; Toussaint et al. , 2006). Specifically, the best end race times have been correlated to the turning times on the second half on the 200 m backstroke (Chatard et al., 2001a), breaststroke (Thomson et al., 2000) and freestyle (Chatard et al., 2001b) events. However, it is unclear if significant changes occur within specific kinematic variables (i.e. underwater distance or velocity) as the race progresses. Until now, most of the research on the turn segment has been focused on kinematic analyses on short distances before and after the wall (between 2.5 and 7.5 m), combined with some dynamic variables regarding the turning push-off. For example, Cossor et al., 1999 used 2.5 m distances, Nicol and Kruger, 1979 and Puel et al., 2012 measured 3 m, whereas Blanksby et al., 1996 and Lyttle et al., 1999 used 5 m. More studies on the underwater phases have been reclaimed to solve some of the questions which have arisen when analyzing the wall contact (Puel et al., 2012) as, in fact, very few studies have analyzed the complete turning movements from stop to stroking restart (Blanksby et al., 2004; Chow et al., 1984, Mason and Cossor, 2001). Additionally, there is a lack of investigations studying the kinematic variables relevant for the backstroke turns (Blanksby et al., 2004; Daniel et al., 2003), specially under competitive conditions (Burkett et al., 2010), despite the fact that backstroke races rely more on the non stroking race segments (Kjendlie et al. , 2006). Therefore, the main goals of this investigation were: 1) to determine the evolution of selected turn variables during competitive backstroke events and 2) to compare these kinematic variables between two different levels of swimmers. It was hypothesized that national level swimmers would reach longer distances into the wall and underwater distances, while obtaining a faster underwater velocity and a shorter 15 m turning time than regional level swimmers.
Sixteen male swimmers who took part in the 200 m backstroke event of the 2007 Spanish Swimming Championships in short course (25 m width x 25 m length) were selected to analyze their turn performances. All experimental procedures were reviewed and approved by the Technical University of Madrid’s Ethics Committee. Written informed consent was obtained from all the participants prior to the commencement of the investigation. All the participants were classified into two groups according to their end race times (n = 29). The swimmers who finished between the 1st and 8th positions were included in the national level group whereas those who finished between the 22nd and 29th positions were included in the regional level group. Table 1 summarizes the descriptive data for both national and regional level groups. Two fixed JVC® GY-DV500E video-cameras recording at 25 Hz, as recommended for swimming race analysis (Arellano et al., 1994), were positioned at the stands, 7 m above and 7 m away from the side of the pool. The cameras optical axes were crossed in order to capture the whole plane delimitated by the water surface; camera 1 captured from the start blocks to 15 m and camera 2 captured from 10 to 25 m. Both cameras were connected to personal computers where the images were stored at real time. The beginning of the time code was provided by a light flash connected to the official timing system and captured by camera 1. Two additional JVC® GV-DV300 video-cameras operating also at 25 Hz were located on the stands (7 m above and 7 m away from the side of the pool) to assist researches in case the contact phase was not visible from the cameras 1 and 2. These cameras were placed with their optical axis at right angles to the long axis of the pool and less than 1 m from the ends of the pool (see Figure 1). The “Individual distances” method was utilized to perform turn analysis using 2D-DLT based algorithms (Abdel-Aziz and Karara, 1971) and the software Photo 23D (Technical University of Madrid, Spain; Cala et al., 2009). Eight pool-side building marks uniformly distributed on the horizontal plane were recorded in each camera and were used as control points for calibration purposes. Selected coordinates of the competitors on the water surface plane during the race were reconstructed from the screen coordinates. The images were synchronized by software from a swum movement (hand entry) simultaneously seen in both cameras between 10 m and 15 m. The beginning of the turn movement was defined by the hand entry of the last stroke on the back (Blanksby et al., 2004), whereas the end of the turn movement was defined by the head emersion, indicating the end of the underwater part (Burkett et al., 2010; Mason and Cossor, 2001). In order to facilitate the interpretation of the turn performance, the stroking phase of each lap was also analyzed from the end of the underwater swim (head emersion) to the beginning (last hand entry on the back) of the turn movements. The following variables were calculated: i) “15 m turn time” (s): the time interval when the swimmer’s head is within 7.5 m before and after the wall; ii) “distance in”: the horizontal distance (m) of the swimmer’s head from the last hand entry on the back to the wall; iii) “underwater distance”: the horizontal distance (m) of the swimmer’s head from the wall to the head emersion; iv) “underwater velocity”: the average velocity (m·s-1) from the beginning of feet contact on the wall to the end of the turn movement; v) “normalized underwater velocity”: the underwater velocity (m·s-1) divided by the mean stroking velocity; and vi) “stroking velocity”: the average velocity (m·s-1) from the end of the underwater swim to the last hand entry on the back in each lap. Thirty-two control points represented by colored buoys from the floating lanes were used to assess the validity of the method. Reference lines connecting the near and far sides of the pool were used to place the colored buoys at exactly a 5 m distance between them. The root mean square error of the 2D-DLT technique was 0.048 m when reconstructing the position of the control points, and 0.044 m when reconstructing the distance between them. To assess the reliability of the method, two technical actions (head emersion and hand entry) were 32 times repeatedly digitalized by the same researcher, with a coefficient of variation less than 1% between the results and no statistical differences (p < 0.05) between them. After a test for the normality of the distribution (Kolmogorov-Smirnov and Shapiro-Wilk), a two-way repeated measures ANOVA was performed to analyze the data using a 2 (swimmers level: national, regional) x 7 (turn segment: first to seventh) design. In the case of detecting any significant interaction, Bonferroni post-hoc tests were performed and effect sizes (ES) as partial eta-squared values were calculated. Also, relationships between the 15 turn time and the various turning phase parameters for each inter-subject group were obtained using Pearson’s correlation coefficients. Significance was set a priori at p < 0.05 for all the statistical tests. All analyses were conducted with the software SPSS 15.0 (SPSS Inc., Chicago, IL, USA).
Distances travelled during the turn movements were 33.9% and 27.4% of the total 200 m backstroke distance for the national and regional level groups, respectively. National level swimmers presented a shorter (p < 0.001) “15 m turn time”, a longer (p < 0.05) “distance in”, a faster (p < 0.05) “underwater velocity” and a faster (p < 0.001) “stroking velocity” than regional level swimmers. The underwater velocity of the national level swimmers was also faster (p < 0.05) than that from the regional level swimmers when normalized to the mean stroking velocity. However, no differences (p = 0.18) were detected between levels for the “underwater distance”. Descriptive values for backstroke turn variables are shown in Table 2. There were no significant correlations (p > 0.05) between the 15 turn time and the various turn phase parameters, except for the underwater distance, which was moderately correlated (r = 0.398; p < 0.05) to the 15 turn time for the regional level group. On the other hand, the 15 m turn time was significantly correlated to the stroking velocity in both national and regional groups of swimmers (r = -0.34; p < 0.05 for national and r = -0.54; p < 0.001 for regional level). The within-subjects turn factor influenced the kinematic turn variables (Wilks lambda = 0.20; F24,284 = 6.986; p < 0.001; ES = 0.99) as the race progressed. For the regional level swimmers, the “15 m turn time” of the first turn was shorter (p < 0.05-0.001) than each one of the following turns, while no temporal differences between turns were found for national level swimmers (Figure 2). For both national and regional level swimmers, “underwater distance” during the last three turns of the race was shorter (p < 0.05-0.001) than that during the first turn segment. The maximal “underwater distance” was obtained during the first turn of the race for both levels (7.38 ± 2.57 m for national and 6.03 ± 1.17 m for regional level) while minimum “underwater distance” was achieved during the fifth (5.96 ± 2.33 m for national level) or the last (4.70 ± 0.86 m for regional level) turn (Figure 3). On the other hand, “distance in” showed no significant differences (p > 0.05) throughout the race for both national and regional groups. National level swimmers presented faster “underwater velocity” values between the last (seventh) and the first and second (p < 0.05), and the fourth turns (p < 0.01). No differences in “underwater velocity” were found throughout the race for the regional level swimmers (Figure 4). Finally, for both national and regional swimmers, “stroking velocity” dropped (p < 0.01) during the first three laps of the race. After that, national level swimmers maintained (p > 0.05) “stroking velocity” until the end of the race, whereas regional level slowed down (p <0.05) during the fourth lap and then maintained (p > 0.05) their “stroking velocity” on the second half of the race. Decrement in “stroking velocity” between the first and last lap of the race were 6.96% and 10.45% for national and regional level swimmers, respectively. Maximal “stroking velocity” was obtained during the first lap of the race (1.60 ± 0.05 m·s-1 for national and 1.48 ± 0.05 for regional level) whereas minimum “stroking velocity” was achieved during the fourth lap for both levels (1.44 ± 0.04 m·s-1 for national and 1.29 ± 0.04 m·s-1 for regional level).
This study examined selected kinematic turn variables during a 200 m backstroke event. To the best of our knowledge, this is the first study that has analyzed the evolution of the turn movements in a backstroke race. Individual turn distances can provide valuable information as very few studies have directly measured distances in competition (Chow et al., 1984; Mason and Cossor, 2001). The contribution of the individual turn segment to the total 200 m race distance (between 27% and 34%) was considerably lower than that estimated from the 15 m turn segment when using fixed distances (52% of the 200 m race). These percentages of individual turn distances indicate that the swimmers observed in the present research swam in apnea at least 50 m of the whole race. “Underwater distance” after the backstroke turn for international level swimmers has been reported to be close to 5 m (Chow et al., 1984). However, our results revealed a longer underwater turn phase as the national swimmers began stroking 6 m away from the turn wall. Considering that national level swimmers could start underwater kicking 3-4 m away from the turning wall (Naemi et al., 2009, Zamparo et al., 2012), it is estimated that they performed 3 to 5 dolphin kicks before they restarted stroking (Zamparo et al., 2012). To date, there are no further studies to which compare this data to as no other investigations have measured turn distances in competition, apart from race analyses conducted by swimming national teams. The competitive level of the swimmers influenced the kinematic turn variables which were examined in this investigation. Differences in “stroking velocity” between national and regional level swimmers (which were close to 10%) could help explaining this data, as it would have an impact on the turn movements. In fact, average stroking velocity was significantly correlated to the “15 m turn time”, whereas no significant correlations were found between the “15 m turn time” and various turn phase parameters. As expected, the best swimmers obtained shorter “15 m turn times” which is in line with findings from previous studies that have employed the fixed distances method (Arellano et al., 1994; Kjendlie et al., 2006; Thomson et al., 2000). However, the best swimmers also obtained faster “underwater velocity” during the 200 m backstroke event, which represents an important finding of the present study. Although the average underwater velocity had been previously correlated to start time (Burkett et al., 2010; Cossor and Mason, 2001; de Jesus et al., 2011; Pereira et al., 2006; Vantorre et al., 2010a; Wakayoshi et al., 1992), no previous studies had identified average underwater turn velocity as a skill-dependent variable. Furthermore, faster underwater velocity of the best swimmers did not seem to depend on their better stroking performance, as differences between swimmers persisted when underwater velocity was normalized to the average stroking velocity. Probably, national level swimmers reached higher “underwater velocity” by generating higher peak forces on the wall (Araujo et al., 2010; Blanksby et al., 1996; Cossor et al., 1999) and by demonstrating a greater underwater kicking efficiency (Zamparo et al., 2012). On the other hand, no “underwater distance” differences were detected between national and regional level swimmers. The great data dispersion due to the “underwater distance” differences between competitors could help to explain this. It could be possible that swimmers extended the turn segment according to their underwater skills and, therefore, it could not be accurate to estimate the same “underwater distance” (i.e. 7.5 m) for all the swimmers. Regarding the distance into the wall, the faster velocities of the best swimmers when approaching the wall (Chow et al., 1984) and their anthropometric characteristics (Pelayo et al., 1996) are in line with the inter-level differences detected in our study. Greater distances to the wall when starting the turning movements have been previously reported as good predictors of better tumble turning times (Blanksby et al., 1996; Puel et al., 2012). The kinematic turn variables did also change along the 200 m backstroke event. Previous studies have reported that the best swimmers have the ability to pace themselves maintining their stroking (Chollet et al., 1997; Letzelter and Freitag, 1983; Toussaint et al., 2006) and turning velocities (Chatard et al., 2001a ; Chatard et al., 2001b ; Thomson et al., 2000). Our results are in line with previous research showing that the national level swimmers maintained their turn time whereas the regional level swimmers increased it in the second half of the race (figure 1). Additionally, the loss in the stroking velocity was greater for regional than for national level swimmers. Taking into consideration that “15 m turn time” included some stroke cycles into the turn segment, it could be expected that the loss in the “stroking velocity” influenced the increase in the turn time of the slowest swimmers. Regarding the various turn phase parameters, our results indicate that the swimmers maintained, or even increased, their underwater velocity throughtout the race. Unlike the “15 m turn time”, the national level swimmers showed the ability to increase the “underwater velocity” in the last turn of the race (Figure 4). This is an important finding, which has not been previously reported, that could partly explain the significant correlations found between the result in a 200 m backstroke event and the performance in the last turn of the race (Chatard et al., 2001a). On the other hand, the turn “underwater distance” did not show the same profile as the “underwater velocity”. For both national and regional level swimmers, the “underwater distance” dropped in the second half of the race probably due to fatigue (Toussaint et al., 2006) and the hypoxic conditions of the underwater phase. These results suggest that the underwater turn phase must be extended only if the velocity in this phase is faster than the stroking velocity (Blanksby et al., 1998; Hubert et al., 2006; Tourny-Chollet et al., 2002) and, therefore, the “underwater distance” must be subordinated to the “underwater velocity”. Finally, the distance into the wall showed a different tendency in comparison to the other turn variables as it did not vary throughout the race. This finding reinforces the assumption that anthropometric characteristics (Chow et al., 1984) or the technical timing of the last strokes before the wall could influence this variable. When evaluating the turn segment in a 200 m backstroke event, the individual distances method can provide additional information in comparison to the traditional “15 m turn time”, as underwater parameters are barely correlated to the 15 m turning times or the stroking skills of the best swimmers (Mason and Cossor, 2001). By presenting the underwater distance and velocity and its evolution throughout the race, the individual distance method could provide support to coaches and swimmers to better define their race strategy and their training programs. For example, the underwater distance and velocity showed a contrary tendency along the seven turn segments. It could be suggested that there is a critical underwater distance for each swimmer where the underwater velocity is maximum in comparison to the stroking velocity and, therefore, the average turn speed could be maximized. Complementarily, according to the percentages of “underwater distance” in this study, coaches are advised to include the underwater swim as an important factor in their training programs for the 200 m backstroke swimmers. For instance, they should put emphasis on the “underwater velocity” to maintain turn proficiency, whereas turn distance could be subordinated to maximize the average velocity. Individual turn variables, where no stroke cycles are included, seem to be a valid tool to enhance turn performance.
In a 200 m backstroke event, the best swimmers obtained a longer distance into the wall, a faster underwater velocity and shorter 15 m turn times. They maintained the turn time throughout the race and increased the underwater velocity at the end of the race. However, they did not travel longer turn distances than regional level swimmers and the turn distance represented a minor quantitative contribution than previously stated. The underwater turn velocity was revealed as a critical variable related to the swimmers’ level of skill, whereas the turn distances seemed to be subordinated in order to maximize the average velocity. The individual variables provided valuable information to evaluate competitive turn performance.
ACKNOWLEDGEMENTS |
The authors would like to thank Javier Mallo Sainz for his valuable help editing the manuscript. |
|
AUTHOR BIOGRAPHY |
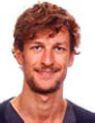 |
Santiago Veiga |
Employment: Associate Professor at the Faculty of Sport Sciences, Technical Madrid University. |
Degree: PhD |
Research interests: Performance analysis in swimming. |
E-mail: Santiago.veiga@upm.es |
|
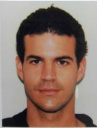 |
Antonio Cala |
Employment: Swimming Biomechanist for High Performance Sport New Zealand |
Degree: PhD |
Research interests: Swimming biomechanics |
E-mail: Antonio.Cala@hpsnz.org.nz |
|
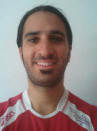 |
Pablo González Frutos |
Employment: Professor at the Francisco de Vitoria University (Athletics, Gymnastics and Sports Training) and is a member of the Sports Biomechanics Laboratory (Technical University of Madrid). |
Degree: MSc |
Research interests: Swimming biomechanics |
E-mail: Antonio.Cala@hpsnz.org.nz |
|
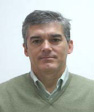 |
Enrique Navarro |
Employment: Director of the Sports Biomechanics Lab. Faculty of Sport Sciences (Universidad Politécnica de Madrid) |
Degree: PhD |
Research interests: Body Mechanical Models (human body and Horse), throwing and kicking skills, swimming, football refereeing and gait analysis. |
E-mail: enrique.navarro@upm.es |
|
|
|
REFERENCES |
 Abdel-Aziz Y.I., Karara H.M. (1971) Proceedings of the ASP Symposium on Close Range Photogrammetry. Direct linear transformation from comparator coordinates into space coordinates in close range photogrammetry.. Falls Church, VA. American Society of Photogrammetry.
|
 Araujo L., Pereira S., Gatti R., Freitas E., Jacomel G., Roesler H., Villas-Boas J. (2010) Analysis of the lateral push-off in the freestyle flip turn.. Journal of Sport Sciences 28, 1175-1181.
|
 Arellano R., Brown P., Cappaert J., Nelson R. (1994) Analysis of 50, 100, and 200 m Freestyle Swimmers at the 1992 Olympic Games.. Journal of Applied Biomechanics 10, 189-199.
|
 Blanksby B., Skender S., Elliott B., McElroy K., Landers G. (2004) An analysis of the rollover backstroke turn by age-group swimmers.. Sports Biomechanics 3, 1-14.
|
 Blanksby B.A., Elliott B.C., McElroy K., Simpson J.R. (1998) Biomechanical factors influencing breaststroke turns by age-group swimmers.. Journal of Applied Biomechanics 14, 180-189.
|
 Blanksby B.A., Gathercole D.G., Marshall R.N. (1996) Force plate and video analysis of the tumble turn by age-group swimmers.. The Journal of Swimming Research 11, 40-45.
|
 Burkett B., Mellifont R., Mason B. (2010) The Influence of swimming start components for selected Olympic and Paralympic swimmers.. Journal of Applied Biomechanics 26, 134-140.
|
 Cala A., Veiga S., García A., Navarro E. (2009) Previous cycling does not affect running efficiency during a triathlon World Cup competition.. Journal of Sport Medicine and Physical Fitness 49, 152-158.
|
 Chatard J.C., Girold S., Cossor J.M., Mason B.R., Blackwell J., Sanders R.H. (2001a) Proceedings of Swim Sessions XIX International Symposium on Biomechanics in Sports. Specific strategy for the medallists versus finalists and semi finalists in the men’s 200 m freestyle at the Sydney Olympic games.. San Francisco. International Society of Biomechanics in Sports.
|
 Chatard J.C., Girold S., Cossor J.M., Mason B.R., Blackwell J., Sanders R.H. (2001b) Proceedings of Swim Sessions XIX International Symposium on Biomechanics in Sports. Specific strategy for the medallists versus finalists and semi finalists in the men’s 200 m freestyle at the Sydney Olympic games.. San Francisco. International Society of Biomechanics in Sports.
|
 Chollet D, Pelayo P., Delaplace C., Tourny C., Sidney M. (1997) Stroking Characteristic Variations in the 100 m Freestyle for male Swimmers of Differing Skill.. Perceptual and Motor Skills 85, 167-177.
|
 Chollet D., Pelayo P., Sidney M., Tourny C. (1996) Comparative analysis of 100m and 200m events in the four strokes in top level swimmers.. Journal of Human Movement Studies 31, 25-37.
|
 Chow J., Hay J., Wilson B., Imel C. (1984) Turning techniques of elite swimmers.. Journal of Sports Sciences 2, 241-255.
|
 Chow J.W., Knudson D.V. (2011) Use of deterministic models in sports and exercise biomechanics research.. Sports Biomechanics 10, 219-233.
|
 Cossor J.M., Blanksby B.A., Elliott B.C. (1999) The Influence of Plyometrlc Training on the Freestyle Tumble Turn.. Journal of Science and Medicine in Sport 2, 106-116.
|
 Cossor J.M., Mason B.R., Blackwell J., Sanders R.H. (2001) Proceedings of Swim Sessions XIX International Symposium on Biomechanics in Sports. Swim start performances at the Sydney 2000 Olympic Games.. San Francisco. International Society of Biomechanics in Sports.
|
 Daniel K., Klauck J., Bieder A. (2003) Biomechanics and medicine in swimming IX-proceedings of the IXth world symposium on biomechanics and medicine in swimming. Kinematic and dynamographic research in different swimming turns.. Saint Etienne, France. University of Saint-Etienne.
|
 de Jesus K., Vilas-Boas J.P., Fernandes R.J., Figueiredo P., Gonçalves P., Pereira S. (2011) Biomechanical analysis of backstroke swimming starts.. International Journal of Sports Medicine 32, 546-551.
|
 Hay J.G. (1985) The biomechanics of sports techniques. Englewood Cliffs, New Jersey. Prentice Hall.
|
 Hubert M., Silveira G.A., Freitas E., Pereira S., Roesler H. (2006) Speed variation analysis before and after the beginning of the stroke in swimming starts.. Portuguese Journal of Sport Sciences 6, 44-45.
|
 Kennedy P., Brown P., Chengalur S.N., Nelson R.C. (1990) Analysis of male and female Olympic swimmers in the 100-meter events.. International Journal of Sport Biomechanics 6, 187-197.
|
 Kjendlie P.S., Haljand R., Fjortoft O., Stallman R.K. (2006) The temporal distribution of race elements in elite swimmers.. Portuguese Journal of Sport Sciences 6, 54-56.
|
 Knudson D. (2009) Significant and meaningful effects in sports biomechanics research.. Sports Biomechanics 8, 96-104.
|
 Letzelter H., Freitag W., Hollander A.P., Huijing P.A., Groot de G. (1983) Biomehanics and medicine in swimming: Proceedings of the Fourth International Symposium of Biomechanics in Swimming and the Fifth International Congress on Swimming Medicine. Stroke length and stroke frequency variations in men’s and women’s 100 m freestyle swimming.. Champaign, IL. Human Kinetics.
|
 Lyttle A.D., Blanksby B.A., Elliott B.C., Lloyd D.G. (1999) Investigating kinetics in the freestyle flip turn push-off.. Journal of Applied Biomechanics 15, 242-252.
|
 Lyttle A.D., Mason B. (1997) A kinematic and kinetic analysis of the freestyle and butterfly turns.. Journal of Swimming Research 12, 7-11.
|
 Mason B.R., Cossor J.M., Blackwell J., Sanders R.H. (2001) Proceedings of Swim Sessions XIX International Symposium on Biomechanics in Sports. Swim turn performances at the Sydney 2000 Olympic Games.. San Francisco. International Society of Biomechanics in Sports.
|
 Miller J., Hay J., Wilson B. (1984) Starting techniques of elite swimmers.. Journal of Sports Sciences 2, 213-223.
|
 Naemi R., Easson W.J., Sanders R.H. (2009) Hidrodynamic glide efficiency in swimming.. Journal of Science and Medicine in Sport 13, 444-451.
|
 Nicol K., Kruger F., Terauds J., Bedingfield E.W. (1979) Swimming III. Impulse exerted in performing several kinds of swimming turns.. Baltimore. University Park Press.
|
 Pelayo P., Sidney M., Kherif T., Chollet D., Tourny C. (1996) Stroking characteristics in freestyle swimming and relationships with anthropometric characteristics.. Journal of Applied Biomechanics 12, 197-206.
|
 Pereira S., Ruschel C., Araujo L.G. (2006) Biomechanical analysis of the underwater phase in swimming starts.. Portuguese Journal of Sport Sciences 6, 79-81.
|
 Prins J.H., Patz A. (2006) The influence of tuck index, depth of foot-plant, and wall contact time on the velocity of push-off in the freestyle flip turn.. Portuguese Journal of Sport Sciences 6, 82-85.
|
 Puel F., Morlier J., Avalos M., Mesnard M., Cid M., Hellard P. (2012) 3D kinematic and dynamic analysis of the front crawl tumble turn in elite male swimmers.. Journal of Biomechanics 45, 510-515.
|
 Seifert L., Payen V., Vantorre J., Chollet D. (2006) The breaststroke start in expert swimmers: a kinematical and coordinative study.. Portuguese Journal of Sport Sciences 6, 90-92.
|
 Shimadzu H., Shibata R., Ohgi Y. (2008) Modelling swimmers’ speeds over the course of a race.. Journal of Biomechanics 41, 549-555.
|
 Thayer A.L., Hay J.G. (1984) Motivating start and turn improvement.. Swimming Technique 20, 17-20.
|
 Thompson K.G., Haljand R., MacLaren D.P. (2000) An analysis of selected kinematic variables in national and elite male and female 100-m and 200-m breaststroke swimmers.. Journal of Sports Sciences 18, 421-431.
|
 Tourny-Chollet C., Chollet C., Hogie S., Papparodopoulos C. (2002) Kinematic analysis of butterfly turns of international and national swimmers.. Journal of Sports Sciences 20, 383-390.
|
 Toussaint H.M., Carol A., Kranenborg H., Truijens M.J. (2006) Effect of fatigue on stroking characteristics in an arms-only 100-m front-crawl race.. Medicine and Science in Sports and Exercise 38, 1635-1642.
|
 Vantorre J., Seifert L., Fernandes R.J., Vilas Boas J.P., Chollet D. (2010a) Kinematical Profiling of the Front Crawl Start.. International Journal of Sports Medicine 31, 16-21.
|
 Vantorre J., Seifert L., Fernandes R.J., Vilas Boas J.P., Chollet D. (2010b) Comparison of Grab Start between Elite and Trained Swimmers.. International Journal of Sports Medicine 31, 887-895.
|
 Wakayoshi K., Nomura T., Takahashi G., Mutoh Y., Miyashita M., McLaren D., Reilly T., Less A. (1992) Biomechanics and medicine in swimming. Swimming Science VI.. Analysis of swimming races in the 1989 Pan Pacific Swimming Championships and 1988 Japanese Olympic trials.. London. Spon Press.
|
 Zamparo P., Vicentini M., Scattolini A., Rigamonti M., Bonifazi M. (2012) The contribution of underwater kicking efficiency in determining “turning performance” in front crawl swimming.. Journal of Sports Medicine and Physical Fitness 52, 457-464.
|
|
|
|
|
|
|