|
|
|
ABSTRACT |
The control of body temperature in Spontaneously Hypertensive Rat (SHR) subjected to exercise in warm environment was investigated. Male SHR and Wistar rats were submitted to moderate exercise in temperate (25°C) and warm (32°C) environments while body and tail skin temperatures, as well as oxygen consumption, were registered. Total time of exercise, workload performed, mechanical efficiency and heat storage were determined. SHR had increased heat production and body temperature at the end of exercise, reduced mechanical efficiency and increased heat storage (p < 0.05). Furthermore, these rats also showed a more intense and faster increase in body temperature during moderate exercise in the warm environment (p < 0.05). The lower mechanical efficiency seen in SHR was closely correlated with their higher body temperature at the point of fatigue in warm environment (p < 0.05). Our results indicate that SHR exhibit significant differences in body temperature control during moderate exercise in warm environment characterized by increased heat production and heat storage during moderate exercise in warm environment. The combination of these responses result in aggravated hyperthermia linked with lower mechanical efficiency. |
Key words:
Thermoregulation, physical exercise, hypertension, heat stress, exercise performance
|
Key
Points
- The practice of physical exercise in warm environment has gained importance in recent decades mainly because of the progressive increases in environmental temperature;
- To the best of our knowledge, these is the first study to analyze body temperature control of SHR during moderate exercise in warm environment;
- SHR showed increased heat production and heat storage that resulted in higher body temperature at the end of exercise;
- SHR showed reduced mechanical efficiency;
- These results demonstrate that when exercising in a warm environment the hypertensive rat exhibit differences in temperature control.
|
The practice of physical exercise, as well as other activities of daily living, in warm environment has gained importance in recent decades mainly because of the progressive increases in environmental temperature, which is directly related to hospitalizations due to cardiovascular disease and heat stroke (Easterling et al., 2000; Ebi et al., 2004; Nybo, 2008). During exercise performed in the heat, hypertensive patients experience an elevated blood pressure response and a greater thermal strain than normotensive individuals (Kenney et al., 1984). Since low-to-moderate exercise is currently prescribed as a non-pharmacological treatment for hypertension (Carneiro-Júnior et al., 2013), and warm environment may represent additional cardiovascular stress during physical activity, the study of body temperature (Tb) control in hypertension emerge as a focus of research. The Spontaneously Hypertensive Rat (SHR) has been extensively used to study cardiovascular disease since it is considered a good animal model of human essential or primary hypertension (Folkow, 1987; Okamoto and Aoki, 1963). Both species share pathophysiological cardiovascular features of hypertension such as increased total peripheral resistance and hyperresponsiveness of the sympathoadrenal system to stressful stimuli (Folkow, 1987; Okamoto and Aoki, 1963; Trippodo and Frohlich, 1981). In order to maintain Tb constant at ~37 °C, the balance between heat production and heat loss is accurately coordinated by the central nervous system (Romanovsky et al., 2007; Webb, 1995). During exercise, the inherent increase in heat production in response to greater metabolic rate leads to the activation of heat loss mechanisms to avoid critically high Tb (Leite et al. 2007; Pires et al., 2007). It is well accepted that homoeothermic animal species are able to control Tb efficiently in a range of cool to moderate ambient conditions (Galloway and Maughan, 1997). However, this capacity has been shown to be impaired during exercise conducted in warm environment (Fuller et al., 1998; Walters et al., 2000). In such situation, increased blood flow to maintain the energy demand of active muscles occurs simultaneously with increases of cutaneous blood flow required to meet the demand of Tb regulation (Folkow, 1987; González-Alonso et al., 2008). These combined demands for blood flow can result in a competition for the available cardiac output (Rowell, 1974), resulting in increased Tb and reduced physical capacity. In fact, it has been shown that high Tb and increased rates of body heating and heat storage are limiting factors for physical performance during prolonged exercise (Fuller et al., 1998; Lacerda et al., 2005; Leite et al., 2006). Taking into account the relationship between the cardiovascular system and the regulation of Tb, and that hypertension can affect the mechanisms of heat dissipation due to increased peripheral resistance (Kellogg et al., 1998; O’Leary and Wang, 1994; Wright et al., 1978), studies have been conducted to investigate the thermoregulatory ability of SHR (Barney et al., 1999; Berkey et al., 1990). Berkey et al. (1990) measured core temperature of SHR at ambient temperatures of 5, 15, 25, 35 and 40°C. After 1h of exposure to these different ambient temperatures the only ambient temperature at which SHR had higher core temperature than normotensive controls was at 40°C (Berkey et al., 1990). Additionally, Barney et al. (1999) exposed SHRs to ambient temperature of 25 or 37.5°C for 3.5h, reporting that SHR had both higher core temperature and evaporative water loss during heat exposure than normotensive controls (Barney et al., 1999). Such studies support that SHR has a hyperesponse to stress, which leads to higher core temperature in situations like exposure to warm environment (Berkey et al., 1990) and dehydration due to heat stress (Barney et al, 1999). Despite these previous results, there are few studies investigating the control of Tb during exercise in warm environment associated with pathological conditions of the cardiovascular system, i.e., hypertension. Thus, the aim of the present study was to investigate temperature control in SHRs subjected to moderate exercise in warm environment.
AnimalsMale SHRs (systolic blood pressure: 170 ± 2.6 mmHg, 300-370 g, n = 8) and normotensive WIS (systolic blood pressure: 104 ± 2.7 mmHg, 350-450 g, n = 8) at 16 weeks of age were individually housed at room temperature of 22 ± 2 °C, under 12/12 h light-dark cycles and had free access to water and rodent chow. Taking into account that the development of hypertension in SHR is dependent on age rather than body weight, age was matched in the present experimental paradigm (Hom et al., 2007). All experimental protocols were approved by the Ethics Committee in Animal Use of our Institution (Protocol #74/2010) and were carried out in accordance with the Guide for the Care and Use of Laboratory Animals (2011).
Implantation of intraperitoneal sensorAnesthesia was induced and maintained with a mixture of ketamine (2 mg·kg-1) and xylazine (2 mg·kg-1, i.p). After pre-surgical preparation of the incision site, a G2 E-Mitter temperature sensor (Mini Mitter, USA) was implanted into the peritoneal cavity through a small incision in the linea alba. Prior to surgery, all rats received a prophylactic dose of antibiotic (Enrofloaxacin, 10 mg·kg-1, i.m.). Postoperative analgesia (Tramadol, 4 mg·kg-1, every 8 h, i.m.) was provided as soon as animals awoke from anesthesia and was continued for 24 hours.
Familiarization periodAfter a 48 h recovery period following surgery, animals were gradually adapted to exercise on a motor-driven treadmill (Panlab, Harvard Apparatus, Spain) by running 5 min per day at 5° inclination for five consecutive days. During the first two days, the running speed was 10 m·min-1, increasing 1 m·min-1 per day. At the third day, animals also started to be familiarized with a skin temperature thermocouple fixed to the tail. This preliminary exercise did not constitute training, but intended to teach them which direction to run (Prímola-Gomes et al., 2007). Electrical stimulation was determined according to each animal’s tolerability (Leite et al., 2012).
Exercise capacity testAfter adaptation, each rat was submitted to an incremental workload running test until the point of fatigue to determine their individual exercise capacity. The test was performed in temperate environment, beginning at a velocity of 10 m·min-1 (5° inclination), with increments of 1 m·min-1 every 3 min until fatigue. Fatigue was defined as the point where animals were no longer able to keep pace with the treadmill for 10 s (Soares et al., 2004).
Experimental protocolOn the day of the experiment and according to the exercise capacity test results, animals were individually submitted to running exercise until fatigue at a constant speed and inclination (60% maximal running speed and 5° inclination). The experimental protocol was performed between 7:00 and 12:00 am and conducted in two ambient temperature situations: 1) temperate environment (TE, 25°C) and 2) warm environment (WE, 32°C). The experimental situations were randomized and balanced. A 48 h interval was allowed for recovery between tests. Intraperitoneal temperature and tail skin temperatures (Ttail) were recorded during moderate exercise. Intraperitoneal temperature was used as an index of body Tb, and measured by an implanted telemetry sensor using the VitalView software package (VitalView, Mini Mitter, USA). Ttail was measured using a thermometer (THR-140, Instrutherm Instruments, Brazil) and a thermocouple (S-09K, Instrutherm Instruments, Brazil) taped with a waterproof adhesive tape to the lateral surface of the tail, 20 mm from the base of the tail (Young and Dawson, 1982). In another experimental setup the metabolic rate was measured by means of oxygen consumption (VO2) with an open-flow indirect calorimeter (Panlab, Harvard Apparatus, Spain), and was continuously recorded during exercise until fatigue using a computerized system (Metabolism, Harvard Apparatus, Spain) (Leite et al., 2007).
CalculationsWorkload (W, kgm) was calculated as follows: body weight (kg) x TTE (min) x treadmill speed (m.min-1) x sinα (treadmill inclination) (Brooks and White, 1978). Mechanical efficiency (ME, %) was calculated using the formula: ME = (workload/energetic cost) x 100 (Brooks et al., 1984). Heat storage (HS, cal) was calculated as HS = (∆Tb) x body weight (g) x c, where ∆Tb represents the change in Tb (Tf–Ti) and Tf and Ti represent the Tb at the fatigue point and prior to exercise, respectively, and c represents specific heat of the body tissues (0.826 cal·g-1·°C-1) (Gordon, 1993). HS was normalized per 100 g of body weight.
Statistical analysisData are reported as mean ± SEM. Two-way ANOVA was used for determining differences between groups and the effect of environment temperature. This was done in order to evaluate the differences in Tb, Ttail and VO2. Significant interactions observed by ANOVA were further evaluated by Tukey post hoc analysis to locate significant differences between means. To evaluate Tb, Ttail and VO2 during experiments compared to baseline we used one-way ANOVA with repeated measures followed by Tukey post hoc. Total time of exercise, workload, mechanical efficiency and heat storage were analyzed by two-way ANOVA followed by the Newman-Keuls post hoc. The correlations were assessed using Pearson’s correlation coefficient. Significance level was set at p <0.05.
During the exercise capacity test, the WIS and SHR showed similar physical performance (47.03 ± 5.06 min WIS vs. 54.21 ± 5.21 min SHR). The maximal running speed was also similar between groups (22.3 ± 0.8 m·min-1 WIS vs. 24.0 ± 0.8 m·min-1 SHR). Thus, the exercise speed corresponding to 60% of maximal running speed was equal between groups (13.4 ± 0.2 m·min-1 WIS vs. 14.4 ± 0.2 m·min-1 SHR). During the moderate exercise protocol, it was not observed differences in physical performance between groups in both temperate (47.03 ± 7.06 min WIS-TE vs. 54.21 ± 5.21 min SHR-TE) and warm environments (15.78 ± 0.95 min WIS-WE vs. 16.96 ± 1.32 min SHR-WE). On the other hand, there was an environment effect during exercise that led to a reduction in physical performance of 66% and 68% for WIS and SHR, respectively (p < 0.05) (Figure 1). Similarly, WIS and SHR had their workload reduced by 69% and 67%, respectively, when comparing the environments. The workload calculated was 25.7 ± 2.67 kgm for WIS-TE, 6.93 ± 0.45 kgm for WIS-WE, 23.04 ± 4.13 kgm for SHR-TE and 8.29 ± 0.75 kgm for SHR-WE. The workload was not different between WIS and SHR groups. As seen in Figure 1, moderate exercise induced an increase in metabolic rate (heat production) for all groups. For all situations, it was not observed any environment effects in heat production. Regardless of the environment, from the 11th until the 17th min of exercise, the SHR group showed greater heat production in comparison to WIS group. Moreover, from the 15th until the 17th min of exercise the SHR-WE had greater heat production in comparison to WIS-WE. As seen in Figure 2A, moderate exercise induced a gradual increase in Tb in all groups. Compared to baseline, WIS-TE, WIS-WE, SHR-TE and SHR-WE had higher Tb values at minutes 7, 6, 7 and 3, respectively (p < 0.05). Related to the environment, animals exercising in warm environment presented higher Tb values from the 4th min until the end of exercise (p < 0.05). Moreover, SHR-WE showed higher Tb compared to SHR-TE after 8 min of exercise (p < 0.05), while WIS-WE was able to maintain its heat balance and showed Tb similar to WIS-TE at the same time. As seen in Fig. 2B, during the moderate exercise Ttail was higher for both groups in warm environment (p < 0.05). As shown in Figure 3A, SHR had lower mechanical efficiency compared to WIS, both in temperate (22.68 ± 2.36% WIS-TE vs. 12.09 ± 0.59% SHR-TE, p < 0.05) and warm environment (24.65 ± 3.21% WIS-WE vs. 14.24 ± 0.99% SHR-WE, p < 0.05). An environmental effect in mechanical efficiency was not observed. A close correlation was found between mechanical efficiency and Tb at the point of fatigue in the warm environment (r = 0.82, p < 0.05) (Figure 3C). To compare the total thermal effect of exercise in both groups, heat storage was calculated (Figure 4). Heat storage in SHR-WE (176.88 ± 25.42 cal/100 g body weight) was higher when compared to WIS-TE (158.12 ± 14.68 cal/100 g body weight), WIS-WE (176.88 ± 25.42 cal/100 g body weight) and SHR-TE (139.00 ± 19.14 cal/100 g body weight) (p < 0.05) (Figure 4).
In the present study, the control of temperature in hypertensive rats subjected to moderate exercise in warm environment was investigated. In this condition, we observed that SHR presented increased heat production and heat storage that resulted in higher Tb at the end of exercise, associated with a reduction in mechanical efficiency. Additionally, a more intense and faster increase in Tb during moderate exercise in the warm environment was shown by SHR. The lower mechanical efficiency seen in SHR was closely correlated with their higher Tb at point of fatigue in warm environment. Taken together, these results demonstrate that when exercising in a warm environment the SHR exhibit differences in temperature control. To maintain heat balance, the heat produced by exercising muscles should be counteracted by increased heat dissipation, otherwise, activity results in greater Tb. The increase in Tb that occurs in response to exercise is the consequence of the increase in metabolic rate coupled with impaired heat dissipation, which does not keep pace with heat production (Webb, 1995). Our results showed that SHR have higher values of Tb at the end of moderate exercise in warm environment. Such response is probably the consequence of higher metabolic heat production shown by SHR, evidenced by a higher VO2 during moderate exercise, associated with no change in heat dissipation through tail vasodilation, as indicated by similar tail temperature. Impaired heat balance in SHR has been well documented in other models of heat stress (Barney et al., 1999; Wright et al., 1977). These studies have shown that when exposed to warm environment, the SHR survival time is shorter in relation to normotensive animals (Wright et al., 1977). Moreover, it has been evidenced that SHR exhibit sympathoadrenal hyperresponsiveness during exposure to cold environment (Kirby et al., 1999), and in condition of dehydration due to heat stress (Barney et al., 1999). Based on measurements of VO2, previous results showed that during continuous exposure to cold heat production was deficient in SHR (Kirby et al., 1999), indicating a possible deficiency in the activation of metabolic heat production in SHR. On the other hand, the regulation of cutaneous sympathetic outflow, which acts to facilitate heat dissipation in response to body heating, is critical during moderate exercise in the heat to maintain Tb within safe limits (Webb, 1995). Therefore, in states of high thermoregulatory demand, the higher sympathetic activity seen in SHR seems to lead to heat intolerance by decreasing heat dissipation through mechanisms of tail vasodilation (Kellogg et al., 1998; O’Leary and Wang, 1994). It is worthy to note that rats dissipate nearly 25% of their resting heat production through vasodilation of the tail skin (Young and Dawson, 1982), and during exercise this percentage may increase to 40% (Shellock and Rubin, 1984). In the current study, Ttail was considered as an index of the tail artery vasomotor tonus, i.e., the magnitude of vasodilation of the tail artery was reflected by tail skin temperature (Leite et al., 2012; Pires et al., 2007; Shellock and Rubin, 1984). Ttail and, hence, cutaneous heat loss are strongly influenced by ambient temperature (Romanovsky et al., 2002). Our results showed that Ttail was already significantly elevated at the beginning of exercise in warm environment. Furthermore, the degree of tail vasodilation reached by both SHR and WIS during exercise in warm environment was higher in comparison to that in temperate environment, indicating amplified blood volume redistribution to the tail for heat dissipation. Nevertheless, Ttail was similar between animals in both environmental temperatures, suggesting equal heat dissipation by this route between strains. However, we cannot overlook the contribution of other routes of heat dissipation, which were not measured in this study, such as the dissipation by the paws and the respiratory tract (Gordon, 1993). In addition, evidence has shown that SHR has a higher ratio of type IIA to I fibers in the soleus muscle, therefore, there is a reduced number of type I fibers inherently more efficient (Damatto et al., 2013; Nagatomo et al., 2009; Novák et al., 2010). These findings can be related to the lower mechanical efficiency exhibited by SHR, which reflects a higher amount of energy to be dissipated as heat. It is recognized that energy efficiency of the body becomes apparent during exercise, when ~20–27% of the energy expended can be used for external work, whereas the remaining energy production is used for homeostasis or dissipated as heat (Brooks et al., 1984). In this sense, SHR had a bigger part of their consumed metabolic energy during exercise to be dissipated as heat. Taking into account that the dissipation of heat from the body is believed to be more important than the control of heat production in the regulation of Tb, particularly during exercise (Lacerda et al., 2005; Leite et al., 2006), such greater demand of heat dissipation may have overridden the limits of tail vasodilation, directly contributing to the higher Tb and increased heat storage observed in SHR-WE. Thus, the overload of heat dissipation capacity as a result of the lower mechanical efficiency may have jeopardized Tb regulation in SHR during exercise in warm environment. It is clear that elevated Tb and increased rates of body heating and heat storage are considered limiting factors that reduce the central nervous system drive for exercise performance and precipitate feelings of fatigue, thus protecting the brain from thermal damage (Fuller et al., 1998; Lacerda et al., 2005; Leite et al., 2006). In the present study, animals exercising in warm environment had reduced total time of exercise and workload. This was probably a consequence of the imposed higher heat load, which augmented Tb after 4 min of exercise until fatigue compared to temperate environment. In addition, the higher demand for heat dissipation seen in these animals may have shifted a larger amount of blood volume to the tail skin, resulting in blood flow competition with active muscles, contributing to the reduced running performance in warm conditions.
In conclusion, the results indicate that hypertensive rats exhibit significant differences in temperature control during moderate exercise in warm environment. The increase in heat production and heat storage during moderate exercise in warm environment resulted in aggravated hyperthermia linked with lower mechanical efficiency.
ACKNOWLEDGEMENTS |
This study was supported by grants from CNPq (Conselho Nacional de Desenvolvimento Científico e Tecnológico), Capes (Coordenação de Aperfeiçoamento de Pessoal de Nível Superior) and FAPEMIG (Fundação de Amparo à Pesquisa do Estado de Minas Gerais). |
|
AUTHOR BIOGRAPHY |
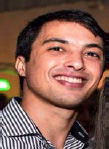 |
Helton O. Campos |
Employment: Graduate student, Federal University of Viçosa |
Degree: MSc |
Research interests: Exercise Physiology, Thermoregulation |
E-mail: heltoncampos@fis-far.dout.ufmg.br |
|
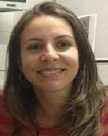 |
Laura H.R. Leite |
Employment: Professor, Federal University of Juiz de Fora |
Degree: PhD |
Research interests: Exercise Physiology, Thermoregulation |
E-mail: laurahrl@gmail.com |
|
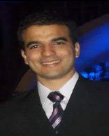 |
Lucas R. Drummond |
Employment: Graduate student, Federal University of Viçosa |
Degree: MSc |
Research interests: Exercise Physiology, Thermoregulation |
E-mail: lucas.drummond@ufv.br |
|
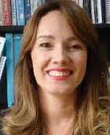 |
Daise N.Q. Cunha |
Employment: Post-Doctoral, Federal University of Viçosa |
Degree: PhD |
Research interests: Cardiovascular Physiology |
E-mail: daisenunes@gmail.com |
|
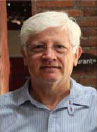 |
Cândido C. Coimbra |
Employment: Professor , Federal University of Minas Gerais |
Degree: PhD |
Research interests: Exercise Physiology, Thermoregulation |
E-mail: coimbrac@icb.ufmg.br |
|
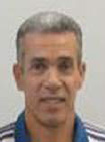 |
Antônio J. Natali |
Employment: Professor, Federal University of Viçosa |
Degree: PhD |
Research interests: Exercise Physiology, Cardiovascular Physiology |
E-mail: anatali@ufv.br |
|
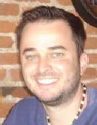 |
Thales N. Prímola-Gomes |
Employment: Professor, Federal University of Viçosa |
Degree: PhD |
Research interests: Exercise Physiology, Thermoregulation |
E-mail: thales.gomes@ufv.br |
|
|
|
REFERENCES |
 Barney C.C., Smith G.L., Folkerts M.M. (1999) Thermal dehydration-induced thirst in spontaneously hypertensive rats. American Journal of Physiology 276, R1302-1310.
|
 Berkey D.L., Meeuwsen K.W., Barney C.C. (1990) Measurements of core temperature in spontaneously hypertensive rats by radiotelemetry. American Journal of Physiology 258, R743-749.
|
 Brooks G.A., Donovan C.M., White T.P. (1984) Estimation of anaerobic energy production and efficiency in rats during exercise. Journal of Applied Physiology: Respiratory Environmental and Exercise Physiology 56, 520-525.
|
 Brooks G.A., White T.P. (1978) Determination of metabolic and heart rate response of rats to treadmill exercise. Journal of Applied Physiology: Respiratory Environmental and Exercise Physiology 45, 1009-1015.
|
 Carneiro-Júnior M.A., Quintão-Júnior J.F., Drummond L.R., Lavorato V.N., Drummond F.R., Cunha D.N., Amadeu M.A., Felix L.B., Oliveira E.M., Cruz J.S., Prímola-Gomes T.N., Mill J.G., Natali A.J. (2013) The benefits of endurance training in cardiomyocyte function in hypertensive rats are reversed within four weeks of detraining. Journal of Molecular and Cellular Cardiology 57, 119-128.
|
 Damatto R.L., Martinez P.F., Lima A.R., Cezar M.D., Campos D.H., Oliveira S.A., Guizoni D.M., Bonomo C., Nakatani B.T., Dal Pai Silva M., Carvalho R.F., Okoshi K., Okoshi M.P. (2013) Heart failure-induced skeletal myopathy in spontaneously hypertensive rats. International Journal of Cardiology 167, 698-703.
|
 Easterling D.R., Meehl G.A., Parmesan C., Changnon S.A., Karl T.R., Mearns L.O. (2000) Climate extremes: observations, modeling, and impacts. Science. 289, 2068-2074.
|
 Ebi K.L., Exuzides K.A., Lau E., Kelsh M., Barnston A. (2004) Weather changes associated with hospitalizations for cardiovascular diseases and stroke in California. International Journal of Biometeorology 49, 48-58.
|
 Folkow B. (1987) Cardiovascular structural adaptation; its role in the initiation and maintenance of primary hypertension. Clinical Science and Molecular Medicine 55, 3s-22s.
|
 Fuller A., Carter R.N., Mitchell D. (1998) Brain and abdominal temperatures at fatigue in rats exercising in the heat. Journal of Applied Physiology 84, 877-883.
|
 Galloway S.D., Maughan R.J. (1997) Effects of ambient temperature on the capacity of perform prolonged cycle exercise in man. Medicine and Science Sports and Exercise 29, 1240-1249.
|
 González-Alonso J., Crandall C.G., Johnson J.M. (2008) The cardiovascular challenge of exercising in the heat. Journal of Physiology 586, 45-53.
|
 Gordon C.J. (1993) Temperature regulation in laboratory rodents.. Cambridge. Cambridge University Press.
|
 Hom S., Fleegal M.A., Egleton R.D., Campos C.R., Hawkins B.T., Davis T.P. (2007) Comparative changes in the blood-brain barrier and cerebral infarction of SHR and WKY rats. American Journal of Physiology: Regulatory, Integrative and Comparative Physiology 292, R1881-92.
|
 Kellogg D.L.J., Morris S.R., Rodriguez S.B., Liu Y., Grossmann M., Stagni G., Shepherd A.M. (1998) Thermoregulatory reflexes and cutaneous active vasodilation during heat stress in hypertensive humans. Journal of Applied Physiology 85, 175-180.
|
 Kenney W.L., Kamon E., Buskirk E.R. (1984) Effect of mild essential hypertension on control of forearm blood flow during exercise in the heat. Journal of Applied Physiology 56, 930-935.
|
 Kirby R.F., Sokoloff G., Perdomo E., Blumberg M.S. (1999) Thermoregulatory and cardiac responses of infant spontaneously hypertensive and Wistar-Kyoto rats to cold exposure. Hypertension 33, 1465-1469.
|
 Lacerda A.C., Marubayashi U., Coimbra C.C. (2005) Nitric oxide pathway is an important modulator of heat loss in rats during exercise. Brain Research Bulletin 67, 110-116.
|
 Leite L.H., Lacerda A.C., Marubayashi U., Coimbra C.C. (2006) Central angiotensin AT1-receptor blockade affects thermoregulation and running performance in rats. American Journal of Physiology: Regulatory, Integrative and Comparative Physiology 291, R603-607.
|
 Leite L.H., Zheng H., Coimbra C.C., Patel K.P. (2012) Contribution of the paraventricular nucleus in autonomic adjustments to heat stress. Experimental Biology and Medicine (Maywood) 237, 570-577.
|
 Leite L.H.R., Lacerda A.C.R., Balthazar C.H., Marubayashi U., Coimbra C.C. (2007) Central AT(1) receptor blockade increases metabolic cost during exercise reducing mechanical efficiency and running performance in rats. Neuropeptides 41, 189-194.
|
 Nagatomo F., Gu N., Fujino H., Takeda I., Tsuda K., Ishihara A. (2009) Skeletal muscle characteristics of rats with obesity, diabetes, hypertension, and hyperlipidemia. Journal of Atherosclerosis and Thrombosis 16, 576-585.
|
 Novák P., Zachařová G., Soukup T. (2010) Individual, age and sex differences in fiber type composition of slow and fast muscles of adult Lewis rats: comparison with other rat strains. Physiological Research 59, 783-801.
|
 Nybo L. (2008) Hyperthermia and fatigue. Journal of Applied Physiology 104, 871-878.
|
 Okamoto K., Aoki K. (1963) Development of a strain of spontaneously hypertensive rats. Japanese Circulation Journal 27, 282-293.
|
 O’Leary D.S., Wang G. (1994) Impaired thermoregulatory cutaneous vasodilation in spontaneously hypertensive rats. Journal of Applied Physiology 77, 692-696.
|
 Pires W., Wanner S.P., La Guardia R.B., Rodrigues L.O., Silveira A.S., Coimbra C.C., Marubayashi U., Lima N.R. (2007) Intracerebroventricular physostigmine enhances blood pressure and heat loss in running rats. Journal of Physiology and Pharmacology 58, 3-17.
|
 Prímola-Gomes T.N., Pires W., Rodrigues L.O., Coimbra C.C., Marubayashi U., Lima N.R. (2007) Activation of the central cholinergic pathway increases post-exercise tail heat loss in rats. Neuroscience Letters 413, 1-5.
|
 Romanovsky A.A. (2007) Thermoregulation: some concepts have changed. Functional architecture of the thermoregulatory system. American Journal of Physiology: Regulatory, Integrative and Comparative Physiology 292, R37-46.
|
 Romanovsky A.A., Ivanov A.I., Shimansky Y.P. (2002) Selected contribution: Ambient temperature for experiments in rats: a new method for determining the zone of thermal neutrality. Journal of Applied Physiology 92, 2667-2679.
|
 Rowell L.B. (1974) Human cardiovascular adjustments to exercise and thermal stress. Physiological Reviews 54, 75-159.
|
 Shellock F.G., Rubin S.A. (1984) Temperature regulation during treadmill exercise in the rat. Journal of Applied Physiology 57, 1872-1877.
|
 Soares D.D., Lima N.R., Coimbra C.C., Marubayashi U. (2004) Intracerebroventricular tryptophan increases heating and heat storage rate in exercising rats. Pharmacology Biochemistry and Behavior 78, 255-261.
|
 Trippodo N.C., Frohlich E.D. (1981) Similarities of genetic (spontaneous) hypertension. Man and rat. Circulation Research 48, 309-319.
|
 Walters T.J., Ryan K.L., Tate L.M., Mason P.A. (2000) Exercise in the heat is limited by a critical internal temperature. Journal of Applied Physiology 89, 799-806.
|
 Webb P. (1995) The physiology of heat regulation. American Journal of Physiology 268, R838-850.
|
 Wright G., Iams S., Knecht E. (1977) Resistance to heat stress in the spontaneously hypertensive rat. Canadian Journal of Physiology and Pharmacology 55, 975-982.
|
 Wright G., Knecht E., Toraason M. (1978) Cardiovascular effects of whole-body heating in spontaneously hypertensive rats. Journal of Applied Physiology 45, 521-527.
|
 Young A.A., Dawson N.J. (1982) Evidence for on-off control of heat dissipation from the tail of the rat. Canadian Journal of Physiology and Pharmacology 60, 392-398.
|
|
|
|
|
|
|