|
|
|
ABSTRACT |
The deceleration sub-phase during the back squat (BSQ) makes it difficult to stimulate the muscles throughout the full range of motion, and it has only been reported for one load during BSQ. The purpose of this study was to investigate whether a deceleration sub-phase occurs during BSQ with different loads and to assess the influence of load on the deceleration sub-phase duration and negative impulse during the deceleration sub-phase. Sixteen healthy men (mean ± standard deviation: age: 25 ± 3 years; height: 1.73 ± 0.07 m; mass: 83.2 ± 16.1 kg; BSQ one repetition maximum (1RM): 163.8 ± 36.6 kg; BSQ 1RM/body weight: 2.0 ± 0.4) who had performed resistance training for at least 1 year were recruited for this study. The subjects performed parallel BSQ with 0%, 12%, 27%, 42%, 56%, 71%, and 85% of each 1RM on a force plate in a random order. The deceleration sub-phase duration and negative impulse during the deceleration sub-phase were calculated from force-time data. The absolute durations of the deceleration sub-phase were not significantly different according to load except for 27% 1RM and 85% 1RM (p = 0.01). However, as the load increased from 12 to 85% 1RM, the relative duration of the deceleration sub-phase decreased (p < 0.05). The negative impulse during the deceleration sub-phase also increased from 0 to 42% 1RM (p < 0.05). A deceleration sub-phase occurs regardless of the load (0%–85% 1RM), and a large portion of the deceleration sub-phase occupied the concentric phase, with low–moderate loads, and a large amount of negative impulse occurred during the short deceleration sub-phase with a high load. |
Key words:
Strength, power, resistance exercise
|
Key
Points
- The deceleration sub-phase occurs during BSQ regardless of the load (0%–85% 1RM).
- A long duration of the deceleration sub-phase occupied the concentric phase with low–moderate loads.
- A large amount of negative impulse occurred during the short deceleration sub-phase with a high load.
|
Lower-body maximal force and the rapid expression of force are important for many athletes, because these factors are related to many types of athletic performance (e.g., jumping). Rapid movements against loads are important to develop lower-body maximal force and the rapid expression of force regardless of whether the load is light or heavy (Behm and Sale, 1993; Fielding et al., 2002). During rapid movements, it is necessary to accelerate the barbell in the first half of the concentric phase, which is known as the “acceleration sub-phase.” However, it is thought that rapid movements during free-weight exercises cause a “deceleration sub-phase” (or sometimes the “decelerating phase”) at the end of the concentric phase (Elliott et al., 1989; Lake et al., 2012; Sanchez-Medina et al., 2010). The acceleration reaches less than zero as a result of the barbell decelerating at the end of the concentric phase. In other words, as force is equal to mass multiplied by acceleration, muscular forces reach less than that in the standing position during the deceleration sub-phase (Sanchez-Medina et al., 2010). Therefore, a deceleration sub-phase during the concentric phase makes it difficult to stimulate muscles throughout the full range of motion. The use of variable resistance training, weightlifting movements, and ballistic training exercises that minimize the deceleration sub-phase and maximize the acceleration sub-phase has been recommended by many sports scientists and coaches (Israetel et al., 2010; Joy et al., 2016; Kawamori and Newton, 2006; Riviere et al., 2017; Stevenson et al., 2010). Previous studies have been based on the assumption that a deceleration sub-phase occurs whether the loads are light or heavy during back squat (BSQ); however, to the best of our knowledge, a deceleration sub-phase has been reported only for one load during BSQ (45% one repetition maximum [1RM]) (Lake et al., 2012). Therefore, it is necessary to investigate the characteristics of the deceleration sub-phase with different loads, including the optimal loads for lower-body maximal force and the rapid expression of force during BSQ, which have a large range (30–70% 1RM) (Soriano et al., 2015). If the loads have different kinetic and kinematic characteristics, the use of this exercise for the development of specific physical characteristics is recommended in order to enhance power. Assuming that a deceleration sub-phase occurs during BSQ with different loads, the duration of the deceleration sub-phase may be expected to increase as the load decreases. As the load decreases, velocity increases (Gonzalez-Badillo and Sanchez-Medina, 2010); thus, a large deceleration may be needed to stop the barbell at the end of the concentric phase during BSQ. On the basis of this assumption, we hypothesized that 1) as the load increases, the absolute duration of the deceleration sub-phase will decrease, and 2) as the load increases, the relative duration of the deceleration sub-phase will decrease. Previous studies have examined the duration of the deceleration sub-phase, but not the magnitude of impulse during the deceleration sub-phase (negative impulse). The negative impulse represents the area under force-time curve (Brady et al., 2017) during deceleration sub-phase. Therefore, we also investigated the negative impulse during the deceleration sub-phase in this study.
Experimental designTo investigate a wide range of loads, subjects performed trials in a random order, with 30%, 40%, 50%, 60%, 70%, 80%, and 90% of the maximal dynamic strength (MDS = 1RM + body mass – [shank mass]) (Cormie et al., 2007) on a force plate. These loads represented 0%, 12%, 27%, 42%, 56%, 71%, and 85% of each subject’s 1RM. The body mass and external load moved together as a unit during BSQ, although the lower leg and feet remain relatively static. Therefore, the loads excluding the mass of the shanks (12% of body mass) were used in this study. Force signals from the force plate were collected, and the deceleration sub-phase duration and sub-phase impulse were calculated from the force signals.
SubjectsSixteen healthy men (powerlifters: n = 6; bodybuilders: n = 2; recreationally trained men: n = 8) (mean ± standard deviation: age: 25 ± 3 years; height: 1.73 ± 0.07 m; mass: 83.2 ± 16.1 kg; BSQ 1RM: 163.8 ± 36.6 kg; BSQ 1RM/body mass: 2.0 ± 0.4) who had performed resistance training for at least 1 year were recruited for this study. All subjects were informed of the experimental procedure, potential risks, and purpose of this study and signed an informed consent document before the investigation. The study was approved by a local Ethical Review Committee on Research with Human Subjects.
ProceduresThe recreationally trained men attended two sessions: a 1RM session was conducted on the first day and a testing session and measurements of height and mass were conducted on the second day. The powerlifters and bodybuilders attended one session, which included measurements of height and mass. The 1RMs of the powerlifters and bodybuilders were determined through the season records. The 1RM session for recreationally trained men was conducted at least a week before the testing session by following the National Strength and Conditioning Association guidelines (McGuigan, 2015; Caufield, 2015). The subjects warmed up for 5 minutes on a cycle ergometer with a workload of 60 Watts. The subjects then performed one set of parallel BSQ for ten repetitions with 50% 1RM and three repetitions with 80% 1RM. After the warm-up, subjects performed parallel BSQ with 0%, 12%, 27%, 42%, 56%, 71%, and 85% of their 1RM on a force plate (PH-6110A; DKH Co., Ltd. Tokyo, Japan) in a random order. The eccentric phase was controlled on the basis of the duration of the phase (about 3 seconds), and the concentric phase was performed by instructing the subject to lift the barbell as fast as possible. The bar placement was in a high-bar position (i.e., high-bar BSQ) and the foot stance was at shoulder width, in accordance with the National Strength and Conditioning Association guidelines (McGuigan, 2015; Caufield, 2015). The subjects were instructed not to try to jump, lift their heels, or pause at the bottom. If these requirements were not met, the trial was repeated again. A 5-minute rest was taken between trials. Force signals from the force plate were collected at 1000 Hz using an A/D converter (Powerlab; ADInstruments, Castle Hill, Australia). The recording software (Chart; Version 5.0 for Windows, ADInstruments, Castle Hill, Australia) was used for recording data. Analysis software (MATLAB; Version 2017a, MathWorks, Natick, MA, USA) was used for analyzing data. The system velocity during BSQ was calculated by integrating the acceleration data from the force-time data using the trapezoid rule, and the period of standing position was defined as the mean ground reaction force for 1 second during the standing position. The threshold for the onset of movement was defined as the point at which the force was reduced by more than 5 standard deviations of the system weight during the period of quiet standing (McMahon et al., 2018; Owen et al., 2014). The start of the concentric phase was defined as the point at which the velocity became positive; that is, a velocity above zero. The end of the concentric phase was defined as the point at which the acceleration changed to zero. The point at which the acceleration became lower than zero was considered the start of the deceleration sub-phase (Elliott et al., 1989; Lake et al., 2012; Sanchez-Medina et al., 2010). The start of concentric phase to the start of deceleration sub-phase was considered part of the acceleration sub-phase. The duration of the deceleration sub-phase was termed “deceleration sub-phase duration,” and the duration of the acceleration sub-phase was termed “acceleration sub-phase duration.” The ‘negative impulse during the deceleration sub-phase’ represents the area under force-time curve (Brady et al., 2017) and was calculated by integrating the force and duration using the tr trapezoid rule. A definition of the deceleration sub-phase during BSQ is shown in Figure 1.
Statistical analysesDescriptive data were expressed as mean ± standard deviation. One-way repeated measures analyses of variance (ANOVA) and a Bonferroni post hoc test were used to determine whether significant differences existed. Statistical significance for all analyses was set at p < 0.05. The η2 values are indicative of effect size (defined as: small < 0.0588, medium < 0.1379, large > 0.1379) (Cohen. 1969). All statistical analyses were performed using a statistical software package (IBM SPSS Statistics, version 24, IBM Corp., Armonk, NY, USA).
The duration of the concentric phase showed a significant, large difference according to the load (one-way ANOVA, p < 0.001, η2 = 0.82). The follow-up Bonferroni post hoc test revealed that as the load increased, the duration of the concentric phase during BSQ increased (p < 0.05). One-way ANOVA and a follow-up Bonferroni post hoc test revealed that the absolute duration of the deceleration sub-phase showed a small but significant difference at 27% 1RM (p < 0.001, η2 = 0.048) and 85% 1RM only (p = 0.01) (Figure 2). Moreover, these tests revealed that as the load increased, large significant increases (p < 0.001, η2 = 0.846) were observed in the acceleration sub-phase duration during BSQ between 12%, 27%, 42%, 56%, 71%, and 85% 1RM (p < 0.05) (Figure 2). One-way ANOVA revealed the relative duration of the deceleration sub-phase to exhibit large significant differences according to the load (p < 0.001, η2 = 0.856). The follow-up Bonferroni post hoc test revealed that as the load increased, the relative duration of the deceleration sub-phase decreased between 12%, 27%, 42%, 56%, 71%, and 85% 1RM (p < 0.05) (Figure 3). The negative impulse during the deceleration sub-phase showed a large significant difference according to the load (one-way ANOVA, p < 0.001, η2 = 0.39). The follow-up Bonferroni post hoc test revealed that as the load increased, the negative impulse during the deceleration sub-phase increased between 0%, 12%, 27%, and 42% 1RM (p < 0.05) (Figure 4).
The results of this study revealed that the deceleration sub-phase occurs during BSQ with different loads and that the relative deceleration sub-phase duration decreased from 12% 1RM to 85% 1RM (approximately 50%-20% of the concentric phase). Although Lake et al. (2012) has not directly mentioned it when defining the end point of the concentric phase by the position of the barbell, the deceleration sub-phase occupied about 30% of the concentric phase during BSQ with 45% 1RM. Although this end point of the concentric phase is different from that in our study, this result is similar to our finding that the deceleration sub-phase occupied about 38% of the duration of the concentric phase during BSQ with 42% 1RM. The relative deceleration sub-phase durations were related to the change in the duration of the concentric phase, but not to the change in the absolute duration of the deceleration sub-phase (Figure 2). These results support our second hypothesis that as the load increases, the relative duration of the deceleration sub-phase decreases. However, these results do not support our first hypothesis that as the load increases, the absolute duration of the deceleration sub-phase decreases. The negative impulse during the deceleration sub-phase significantly increased from 0% 1RM to 42% 1RM as a result of change in force production. Considering these results, the deceleration sub-phase occurs during BSQ with the optimal load (30–70% 1RM) for lower-body maximal force and the rapid expression of force. The characteristics of the deceleration sub-phase were different according to the loads; a large portion of the deceleration sub-phase occupied the concentric phase with low–moderate loads and a large amount of negative impulse occurred during the short deceleration sub-phase with a high load. The actual movement velocity is one of the important factors in developing lower-body maximal force and the rapid expression of force, and comparably low to moderate loads are used during BSQ. However, a large portion of the deceleration sub-phase occupies the concentric phase, and the acceleration sub-phase is short with comparably low to moderate loads. Therefore, it is important to do exercises that have a long acceleration sub-phase. Lake et al. (2012) reported that the acceleration sub-phase during ballistic exercises (i.e., jump squat with 45% 1RM) is 8.6% longer than during non-ballistic exercises (i.e., squat with 45% 1RM). Moir et al. (2012) reported that external power and knee joint power are highest at 0% 1RM and that hip joint power is highest at 42% 1RM. However, as the loads increased more than 42% 1RM, the external power and joint power are decreased and movement velocities also might be decreased. The greatest peak power during high pull occurs with a comparably light-to-moderate load (Suchomel et al., 2014). Therefore, ballistic exercises (e.g., jump squat) and high pull might be more effective exercises to overcome the negative effect of the deceleration phase if athletes tend to develop lower-body maximal force and the rapid expression of force with light-to-moderate load. The lower-body maximal force and the rapid expression of force develops as long as subjects attempt to move rapidly regardless of the actual velocity for subjects who have a low-strength level (Behm and Sale, 1993; Kawamori and Newton, 2006). The intention to move rapidly with a high load during BSQ generates a longer acceleration sub-phase so that the negative effect of the deceleration sub-phase is superficially small. However, the present study revealed that a large amount of negative impulse occurs during the short deceleration sub-phase with a high load; thus, it is also important to overcome the negative effect of the deceleration sub-phase with a high load. Rapid movements against heavy loads that are necessary for developing athletic performance occur during some of the weightlifting movements. Moreover, it could be that weightlifting movements generate a longer acceleration sub-phase than does nonballistic exercise (Suchomel et al., 2017; Suchomel and Sole, 2017). The system (body and barbell) peak power and body peak power have been shown to be highest at 80% and 90% 1RM, respectively, during power clean (McBride et al., 2011). The peak power output during hang power clean has shown to be highest at 70%-80% 1RM (Kawamori et al., 2005; Kilduff et al., 2007). Regarding other weightlifting movements, Comfort et al. (2012) advocated that if the goal is maximizing force production, then midthigh clean pull at higher load (140% 1RM) might be advantageous. Therefore, weightlifting movements and weightlifting derivatives with high loads might be more effective exercises to overcome the negative effect of the deceleration sub-phase. It is also expected that variable resistance training (e.g., band squat, chain squat) overcomes the negative effect of the deceleration sub-phase (Israetel et al., 2010; Joy et al., 2016; Riviere et al., 2017; Stevenson et al., 2010). However, little is known about the influence of variable resistance on the deceleration sub-phase. Future studies on whether variable resistance training could overcome the negative effect of the deceleration phase or not are needed. In summary, ballistic exercise and high pull with light-to-moderate load and weightlifting movements (e.g., power clean, hang power clean, and midthigh clean pull) with a high load might be more effective exercises to overcome the negative effect of the deceleration sub-phase. The study had some limitations. First, the cross-sectional design prevented any conclusions about the effects of long-term resistance training on the deceleration sub-phase during BSQ and athletic performance. The sample size was slightly small; therefore, while significant differences were observed in some analyses, other results may have been influenced by the sample size. The findings that the duration of the deceleration sub-phase does not change according to the load and that the relative duration of the deceleration sub-phase was largely influenced by the load are supported by the size of the effect observed. The effect size on the negative impulse during the deceleration sub-phase was also large, indicating that this parameter was not influenced by the small sample size. In the current study, three groups of men were recruited, with similar but slightly different exercise characteristics (powerlifters, bodybuilders, and recreationally trained men). Although the results did not show large differences between the three groups, the current results can only be applied to trained men.
The deceleration sub-phase occurs during BSQ regardless of whether the loads are light or heavy, and the absolute duration of the deceleration sub-phase do not change according to the load. However, as the load increased, the relative duration of the deceleration sub-phase significantly decreased, and the sub-phase negative impulse significantly increased from light to moderate load.
ACKNOWLEDGEMENTS |
The authors are grateful to the participants in this study. This paper is a part of the research performed under a Waseda University Grant for Special Research Projects (Project number: 2017K-315). The reported experiments comply with the current laws of the country, in which they were performed. The authors have no conflicts of interests to declare. |
|
AUTHOR BIOGRAPHY |
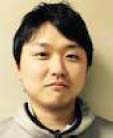 |
Takafumi Kubo |
Employment: Graduate School of Sport Sciences, Waseda University |
Degree: MSc |
Research interests: Strength and conditioning |
E-mail: t.k-52-waseda@ruri.waseda.jp |
|
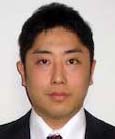 |
Kuniaki Hirayama |
Employment: Faculty of Sport Sciences, Waseda University |
Degree: PhD |
Research interests: Strength and conditioning |
E-mail: k.hirayama@waseda.jp |
|
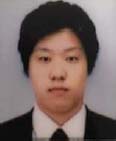 |
Nobuhiro Nakamura |
Employment: Graduate School of Sport Sciences, Waseda University |
Degree: MSc |
Research interests: Exercise physiology |
E-mail: nobu0902@toki.waseda.jp |
|
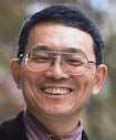 |
Mitsuru Higuchi |
Employment: Faculty of Sport Sciences, Waseda University |
Degree: PhD |
Research interests: Exercise physiology |
E-mail: mhiguchi@waseda.jp |
|
|
|
REFERENCES |
 Behm D.G., Sale D.G. (1993) Intended rather than actual movement velocity determines velocity-specific training response. Journal of Applied Physiology 74, 359-368.
|
 Brady C., Comyns T., Harrison A., Warrington G. (2017) Focus of Attention for Diagnostic Testing of the Force-Velocity Curve. Strength and Conditioning Journal 39, 57-70.
|
 Caulfield, S (2015) Exercise Technique for Free-Weight and Machine
Training. In: Essentials of Strength Training and Conditioning.
Eds: Haff, G.G. and Triplett, N.T. United States of America:
Human Kinetics. National Strength and Conditioning
Association. 4th edition. 351-408.
|
 Cohen J. (1969) Statistical power analysis for the behavioral sciences.
1
st Edition. Cambridge, New York: Academic Press.
|
 Comfort P., Udall R., Jones P.A. (2012) The effect of loading on kinematic and kinetic variables during the midthigh clean pull. Journal of Strength and Conditioning Research 26, 1208-1214.
|
 Cormie P., McCaulley G.O., Triplett N.T., McBride J.M. (2007) Optimal loading for maximal power output during lower-body resistance exercises. Medicine and Science in Sports and Exercise 39, 340-349.
|
 Elliott B.C., Wilson G.J., Kerr G.K. (1989) A biomechanical analysis of the sticking region in the bench press. Medicine and Science in Sports and Exercise 21, 450-462.
|
 Fielding R.A., LeBrasseur N.K., Cuoco A., Bean J., Mizer K., Fiatarone Singh M.A. (2002) High-velocity resistance training increases skeletal muscle peak power in older women. Journal of American Geriatrics Society 50, 655-662.
|
 Gonzalez-Badillo J.J., Sanchez-Medina L. (2010) Movement velocity as a measure of loading intensity in resistance training. International Journal of Sports Medicine 31, 347-352.
|
 Israetel M.A., McBride J.M., Nuzzo J.L., Skinner J.W., Dayne A.M. (2010) Kinetic and kinematic differences between squats performed with and without elastic bands. Journal of Strength and Conditioning Research 24, 190-194.
|
 Joy J.M., Lowery R.P., Oliveira de Souza E., Wilson J.M. (2016) Elastic Bands as a Component of Periodized Resistance Training. Journal of Strength and Conditioning Research 30, 2100-2106.
|
 Kawamori N., Crum A.J., Blumert P.A., Kulik J.R., Childers J.T., Wood J.A., Stone M.H., Haff G.G. (2005) Influence of different relative intensities on power output during the hang power clean: identification of the optimal load. Journal of Strength and Conditioning Research 19, 698-708.
|
 Kawamori N., Newton R.U. (2006) Velocity specificity of resistance training: actual movement velocity versus intention to move explosively. Strength and Conditioning Journal 28, 86.
|
 Kilduff L.P., Bevan H., Owen N.I.C., Kingsley M., Bunce P., Bennett M., Cunningham D. (2007) Optimal loading for peak power output during the hang power clean in professional rugby players. International Journal of Sports Physiology and Performance 2, 260-269.
|
 Lake J., Lauder M., Smith N., Shorter K. (2012) A Comparison of Ballistic and Nonballistic Lower-Body Resistance Exercise and the Methods Used to Identify Their Positive Lifting Phases. Journal of Applied Biomechanics 28, 431-437.
|
 McBride J.M., Haines T.L., Kirby T.J. (2011) Effect of loading on peak power of the bar, body, and system during power cleans, squats, and jump squats. Journal of Sports Science 29, 1215-1221.
|
 McGuigan, M.R. (2015) Administration, Scoring, and Interpretation of
Selected Tests. In: Essentials of Strength Training and
Conditioning. Eds: Haff, G.G. and Triplett, N.T. United States
of America: Human Kinetics. National Strength and
Conditioning Association. 4th edition. 259-316.
|
 McMahon, J.J., Suchomel, T.J., Lake, J.P. and Comfort, P. (2018)
Relationship between reactive strength index variants in rugby
league players. Journal of Strength and Conditioning Research,
Feb 1.
|
 Moir G.L., Gollie J.M., Davis S.E., Guers J.J., Witmer C.A. (2012) The effects of load on system and lower-body joint kinetics during jump squats. Sports Biomechanics 11, 492-506.
|
 Owen N.J., Watkins J., Kilduff L.P., Bevan H.R., Bennett M.A. (2014) Development of a criterion method to determine peak mechanical power output in a countermovement jump. Journal of Strength and Conditioning Research 28, 1552-1558.
|
 Riviere M., Louit L., Strokosch A., Seitz L.B. (2017) Variable resistance training promotes greater strength and power adaptations than traditional resistance training in elite youth rugby league players. Journal of Strength and Conditioning Research 31, 947-955.
|
 Sanchez-Medina L., Perez C.E., Gonzalez-Badillo J.J. (2010) Importance of the propulsive phase in strength assessment. International Journal of Sports Medicine 31, 123-129.
|
 Soriano M.A., Jimenez-Reyes P., Rhea M.R., Marin P.J. (2015) The optimal load for maximal power production during lower-body resistance exercises: a meta-analysis. Sports Medicine 45, 1191-1205.
|
 Stevenson M.W., Warpeha J.M., Dietz C.C., Giveans R.M., Erdman A.G. (2010) Acute effects of elastic bands during the free-weight barbell back squat exercise on velocity, power, and force production. Journal of Strength and Conditioning Research 24, 2944-2954.
|
 Suchomel T.J., Lake J.P., Comfort P. (2017) Load absorption force-time characteristics following the second pull of weightlifting derivatives. Journal of Strength and Conditioning Research 31, 1644-1652.
|
 Suchomel T.J., Sole C.J. (2017) Force-Time-curve comparison between weight-lifting derivatives. International Journal of Sports Physiology and Performance 12, 431-439.
|
 Suchomel T.J., Wright G.A., Kernozek T.W., Kline D.E. (2014) Kinetic comparison of the power development between power clean variations. Journal of Strength and Conditioning Research 28, 350-360.
|
|
|
|
|
|
|