|
|
|
ABSTRACT |
Blood flow restriction (BFR) training has been shown to induce favorable changes in muscle mass and strength with a considerably low training load (20 – 30% 1RM). However, it has never been evaluated if an additional post-exercise protein supplementation enhances the effects of this training regimen. Thirty healthy older men (60.1 ± 7.6 years) were enrolled in the 8-week intervention and randomly allocated to one of the following groups: low-load BFR training with protein (collagen hydrolysate) supplementation (BFR-CH), low-load BFR training with placebo (BFR-PLA), or a control group without training, but with protein supplementation (CON). Muscle cross-sectional area (CSA), muscle strength, circulating reactive oxygen species and IGF-1 were measured before and after the intervention. Muscle CSA increased in both BFR-CH and BFR-PLA groups by 6.7 ± 3.2 % (p < 0.001) and 5.7 ± 2.7 % (p < 0.001) respectively. No significant changes were observed in the CON group (1.1 ± 1.7 %, p = 0.124). Evaluation of isometric strength (p = 0.247), insulin-like growth factor 1 (p = 0.705) and the production of reactive oxygen species (pt1 = 0.229; pt2 = 0.741) revealed no significant interaction effect but a significant long-term time effect (p < 0.001). Our results demonstrate that BFR training is an effective alternative for increasing muscle CSA in older men. Although there was a trend towards greater muscle mass adaptations in the BFR-CH group, these findings showed no statistical significance. Further research with larger sample sizes is needed to confirm these results. |
Key words:
Blood flow restriction, sarcopenia, protein supplementation, muscular hypertrophy, magnetic resonance imaging, aging
|
Key
Points
- Blood flow restriction training is an effective training strategy for increasing muscle adaptations in older people.
- The addition of collagen hydrolysate demonstrated a positive trend towards higher increases in muscle mass and strength but did not reach statistical significance.
- Further studies are needed to verify these effects with bigger sample sizes.
|
According to health surveys, between 20 – 50% of older adults in Europe and the USA report difficulties with activities of daily living (Heikkinen, 2008; Manton and Gu, 2001). One of the factors that contribute to this loss of functional capacity and mobility in older age is the progressive decline of skeletal muscle mass with increasing age (English and Paddon-Jones, 2010; Forbes and Reina, 1970). The presence of both low muscle mass and muscle strength is described by the syndrome of sarcopenia (Abellan van Kan et al., 2012; Cruz-Jentoft et al., 2010). The consequences of sarcopenia are often severe and can result in decreased postural control (Wolfson et al., 1995), increased incidence of falls (Moreland et al., 2004) and deficits in quality of life (Tsekoura et al., 2017) in older adults. Furthermore, the progressive loss of muscle mass during the aging process is accompanied by an increase in cardiometabolic risk factors leading to a higher incidence of type 2 diabetes or atherothrombotic diseases (Sayer et al., 2013). To maximize the period of effective functioning in older age, Cruz-Jentoft and Landi (2014) recommend comprehensive multidimensional approaches combining nutrition and physical exercise as main components of sarcopenia interventions. Concomitantly, common exercise guidelines aiming to increase muscle cross-sectional area (CSA) recommend using moderate to high training loads of 70 – 85% of individuals’ one-repetition maximum (1RM) (ACSM, 2009). However, in a clinical setting and especially for older people with comorbidities, training with such heavy loads is often contraindicated or impossible (Gheno et al., 2012), due to comorbidities such as coronary heart diseases or joint and muscle impairments. Findings from previous studies have indicated that low-intensity resistance exercise in combination with blood flow restriction (BFR) promotes muscle mass increases similar to what is seen after high-load training with 80% 1RM (Vechin et al., 2015). Fry et al. (2010) have demonstrated that BFR training enhances protein synthesis by phosphorylating the mammalian target of rapamycin (mTOR) and its downstream effectors p70 ribosomal S6 kinase (S6K1) in older men. S6K1 has repeatedly been shown to regulate mRNA translation initiation and to be involved in training-induced hypertrophy (Baar and Esser, 1999). In parallel, evidence suggests that ingesting dietary protein additionally enhances the effects of prolonged resistance training on muscle mass and strength in younger and older people (Cermak et al., 2012). By activating the mTOR signaling pathway and thus protein synthesis, it is believed that branched chain amino acids (BCAAs) or di- and tripeptides such as hydroxyprolyl-glycine (Hyp-Gly) stimulate muscular hypertrophy (Cermak et al., 2012; Kitakaze et al., 2016). Previous studies showed that considerable amounts of Hyp-Gly have been found in human blood after the intake of collagen hydrolysate (CH) (Shigemura et al., 2011). Recent investigations in this field have also demonstrated that the daily ingestion of 15 g collagen peptides (CP) is an effective strategy for improving fat free mass and strength following high-load resistance training in older men (Zdzieblik et al., 2015). Therefore, the purpose of this study was to examine the influence of BFR training with collagen hydrolysate supplementation on muscle mass and function in older men at risk of sarcopenia. We hypothesized that this training method is a promising alternative in order to counteract the age-related decline in muscle mass and is therefore beneficial for populations who are not capable of lifting near-maximum loads. Additionally, reactive oxygen species (ROS) and insulin-like growth factor-1 (IGF-1) were measured, since both play crucial roles in muscular remodeling and promote cellular adaptations (Powers et al., 2010). As a second aim, we wanted to determine whether a collagen hydrolysate supplementation enhances the effects of this training modality.
ParticipantsThirty-nine healthy older men aged 50 years or older were recruited to participate in this study. All participants were apparently healthy with a regular physical activity level being less than 60 minutes per week assessed with the Freiburg Questionnaire of physical activity (Frey et al., 1999). Exclusion criteria included the presence of a chronic illness, history of deep vein thrombosis, uncontrolled hypertension, kidney failure, cardiovascular diseases and smoking. Additionally, participants with acutely elevated D-dimer concentrations (> 0.5 mg/l) or intolerance against collagen hydrolysate or silicon dioxide were not included. All participants were advised not to change their regular physical activity or eating behaviors during the intervention. Two participants did not meet the inclusion criteria due to regular smoking and elevated D-dimer concentrations and were thus excluded before assignment to any of the groups. The study was approved by the local ethics committee of the University of Freiburg (356/17) and conducted in accordance with the Declaration of Helsinki. Experimental procedures and potential risks were explained before informed consent was obtained prior to participation. A total of 30 participants completed the investigation and none of the dropouts was due to side effects of training.
Study designA prospective, randomized, placebo-controlled design was implemented. Before the 8-week intervention period, the participants were randomly allocated into one of the following three groups: BFR training with collagen supplementation (BFR-CH), BFR training with placebo (BFR-PLA) or a group that had no training but collagen supplementation only (CON). Allocation sequence was concealed in order to prevent selection bias (Schulz et al., 2010). Both training groups and all therapists and outcome assessors were blinded, whereas the CON group was not blinded. One week prior to the start of the intervention, all participants were screened using a comprehensive anamnesis screen, physical examination, as well as blood draws for the measurement of safety parameters such as hemogram, creatinine, urea, aspartate transaminase, alanine aminotransferase and D-dimer. Furthermore, isometric leg strength, metabolic parameters, and thigh muscle cross-sectional measured by magnetic resonance imaging (MRI) were determined within one week before and after the intervention.
TrainingDuring the 8-week intervention, the BFR-CH and BFR-PLA groups completed a lower extremity training program on three days of the week, with at least one day rest between consecutive sessions. For all these participants, a training session consisted of one set of 45° leg press exercise (FREI AG, Kirchzarten, Germany) with 30 repetitions at 20% of their individual 1RM, followed by three sets of 15 repetitions with 30s inter-set rest periods. This is a common low-intensity BFR exercise protocol reported in recent research (Vechin et al., 2015). Additional 1RM tests were implemented every two weeks (6 sessions) to progressively adjust training load to the current strength level of each individual. At mid-point of the intervention period (after week 4, 12 sessions) the training load was increased from 20% 1RM to 30% 1RM. A metronome was used during each session to ensure that the participants held a tempo of 2 seconds for concentric and 2 seconds for eccentric muscle action. Arterial occlusion pressure (AOP) for each participant was recorded in a sitting position from both legs with a 12-cm-wide pneumatic nylon tourniquet (Zimmer Biomet, Warsaw, Indiana, USA) applied at the most proximal portion of each thigh. This cuff position corresponded to the training position for the cuff. AOP was calculated after the cuff pressure was successively increased until a pulse was no longer detected at the posterior tibial artery by Doppler ultrasound (Handydop, Kranzbühler, Solingen, Germany). At this point, an arterial occlusion of 100% was assumed. The same pneumatic nylon tourniquet was used for all BFR training sessions and kept inflated by a computerized tourniquet system (A.T.S. 3000, Zimmer Biomet, Warsaw, IN, USA) at 50% of each individual’s AOP during the entire session including rest periods. To consider the participants’ current blood pressure, this AOP determination method was conducted immediately before every training session. All the prescribed repetitions per set were successfully completed by all individuals.
NutritionAll participants were instructed to maintain their dietary habits during the intervention period. Nutritional status was examined using Nutriguide 4.6 Software (Nutri Science GmbH, Hausach, Germany). Participants recorded their dietary habits during three consecutive days, including two weekdays and one day on the weekend before and after the intervention. Additionally, the participants of the groups BFR-CH and CON received a daily dose of 15 g of collagen hydrolysate (Collagen Research Institute, Kiel, Germany), whereas group BFR-PLA received a placebo supplement (silicon dioxide) being similar in taste and appearance. Both specimens were dissolved in 250 ml of water and consumed within 60 minutes after completion of each training session. On non-training days, the supplements were consumed at the same time of the day as on training days.
Thigh muscle Cross-Sectional Area (CSA)Thigh muscle CSA was obtained through MRI scanning (Magnetom, Aera 1.5T, Siemens, Berlin, Germany). Subjects were positioned in a supine position with knees extended and legs straight. A T1-weighted, turbo spin-echo, axial plane sequence was performed with a repetition time of 544 ms and an echo time of 9.9 ms. Three scans with a slice thickness of 1.0 cm were acquired at 50% of the femur length (measured from the greater trochanter to the inferior border of the lateral epicondyle of the femur) for analyses. The images were then transferred to a personal computer and muscle tissue CSA of each image was determined with image-analysis software (ImageJ 1.51, NIH, Maryland, USA). Subsequently, the mean values of the three images were used for calculation of muscle CSA. This procedure was performed on both legs and the average of both legs was used for determination of total thigh muscle cross-sectional area. The coefficient of variation between measurements was 1.54%.
Muscular strengthIsometric 1RM was analyzed via a 90° leg press device constructed by the University of Freiburg, Germany. After a warm-up of three submaximal contractions, the participants were instructed to perform three maximum voluntary contractions (MVC). Each attempt was separated by a four-minute resting period to ensure adequate recovery. The highest MVC value was used for data analysis. As mentioned earlier, dynamic leg strength was determined on a leg press machine (45° leg press, FREI AG, Kirchzarten, Germany) before and every two weeks during the intervention to provide a progressive increase of the exercise intensity. Before actual testing, a specific warm-up with two sets of ten repetitions with submaximal load was completed. After a two minute resting period, participants performed two more warm-up sets with a load allowing three to five repetitions (Baechle and Earle, 2000). Subsequently, the actual 1RM-test was performed with a full range of motion reaching from a 90° knee angle to the knee being almost but not fully extended. Following each successful lift, the load was increased by 5-10% until the participant failed to lift the load with proper technique through the entire range of motion (Baechle and Earle, 2000). Single attempts were separated by a four-minute rest period to ensure adequate recovery. Final 1RM of the leg press exercise was determined as the maximum load lifted through full range of motion and was detected in less than five 1RM attempts.
Metabolic parametersReactive Oxygen Species (ROS): Total ROS production was analyzed before and immediately after the first and last training session from a capillary blood sample. Therefore, this parameter was determined only in the training groups (BFR-CH and BFR-PLA). The production of ROS was measured by electron paramagnetic resonance (EPR) imaging. A benchtop EPR-spectrometer [E-Scan M, Bruker, Germany] was equipped with a temperature controller BIO-III to measure ROS production under in vivo conditions at 37°C. Total ROS production was analyzed from 20 µl of capillary blood, sampled directly from the right index fingertip of each participant using a standard Eppendorf pipette. After adding 20 µl of oxygen-sensitive label (NOX 15.1-5 µmol/l) to the 20 µl blood sample in a micro centrifuge tube, the mixture was vortexed and placed on ice. Utilization of this oxygen-sensitive label allows monitoring of cellular oxygen consumption (Mariappan et al., 2009). Subsequently, 15 µl of the blood-label solution was combined with 15 µl of Krebs-HEPES buffer diluted spin probe 1-hydroxy-3-methoxycarbonyl-2,2,5,5-tetramethylpyrrolidine (CMH, 400 µmol/l), obtained from Noxygen Science Transfer and Diagnostics GmbH [Elzach, Germany]. Under these circumstances, released ROS in capillary blood interacts in the intracellular and extracellular space with CMH to form the stable and detectable radical CM° (Mrakic-Sposta et al., 2012). The following settings were used for the bench-top EPR-spectrometer: center field: g = 2.011, sweep width: 60 G, frequency = 9.76 GHz, power = 20.97 mW, gain: 1 x 10³, modulation amplitude: 1.06 G, sweep time: 5.24 sec, number of scans: 10. Calibration of EPR signal was performed using a standard concentration of CM° (10 µmol/l) filled into a 50 µl glass capillary. The instrument’s coefficient of variation is 2 – 5%. Insulin-Like Growth Factor 1: Systemic IGF-1 in venous blood serum was determined before and after the 8-week intervention in all participants using a chemiluminescence immunoassay.
Statistical analysisThe statistical software package SPSS version 24.0 (IBM, Armonk, USA) was used for all analyses with an alpha level of p < 0.05. Normal distribution of all data was checked for all variables using Kolmogorov-Smirnov test. Interaction effects were tested with repeated measures ANOVA (RMANOVA). For further examination of significant interactions, post hoc paired t-tests (within each group) were performed. To correct for multiple comparisons, Bonferroni correction was applied, and the significance level subsequently adjusted to p < 0.017.
Baseline participant characteristics are presented in Table 1. The following descriptive parameters are reported as mean ± standard deviation, as well as percentage changes [((post value – pre value) / pre value) · 100]. Baseline values among the three experimental groups for age, anthropometric variables, muscular strength and muscle CSA were not statistically different (p > 0.05).
Muscle CSAThe muscle CSA of the thigh significantly increased by 6.7 ± 3.2 % (p < 0.001, 95% CI: 4.5 – 8.9) and 5.7 ± 2.7 % (p < 0.001, 95% CI: 3.9 – 7.5) in the BFR-CH and BFR-PLA groups, respectively. No significant change (p = 0.124, 95% CI: -0.3 – 2.6) was observed in the CON group (+ 1.1 ± 1.7 %). After calculation of a two-way RMANOVA both main effect of time (p < 0.001, ηp² = 0.760) and interaction effect reached statistical significance (p < 0.001, ηp² = 0.448) with no significant difference between BFR-CH and BFR-PLA conditions (Figure 1).
Muscular strengthLooking at the relative changes (Figure 2), the BFR-CH group had a 10.2 ± 24.8 % (95% CI: -6.5 – 26.8) increase of strength from pre- to post-intervention, whereas the group BFR-PLA showed increases of 4.8 ± 11.4 % (95% CI: -2.9 – 12.5). In the CON group, isometric strength decreased by 5.3 ± 8.6% (95% CI: -12.5 – 1.9) (Figure 2). However, the inferential analysis of isometric leg strength revealed neither a significant main effect of time (p = 0.425, ηp² = 0.024) nor a significant interaction (p = 0.247, ηp² = 0.098).
Metabolic parametersSerum levels of insulin-like growth factor 1 (IGF-1) increased after the intervention by 15.3 ± 41.4 µg/l (95% CI: -12.6 – 43.1) in the BFR-CH group and 10.6 ± 9.7 µg/l (95% CI: 4.1 – 17.1) in the BFR-PLA group. Elevations of 5.0 ± 8.7 µg/l (95% CI: -2.3 – 12.3) were observed in the CON group. Statistical analyses revealed a significant time effect (p < 0.05, ηp² = 0.143) but no significant interaction effect (p = 0.705, ηp² = 0.026). Total ROS production decreased from pre- to immediately post-exercise (first training session) by -0.009 ± 0.143 µmol/l/min (95% CI: -0.105 – 0.087) in the BFR-CH group but increased in the BFR-PLA group (0.056 ± 0.100 µmol/l/min; 95% CI: -2.9 – 12.5). However, these results were not associated with a significant main effect of time (p = 0.383, ηp² = 0.038) nor interaction (p = 0.229, ηp² = 0.072). After the 8-week intervention, the resting ROS production decreased in the BFR-CH group by -22.2 ± 20.0 % (95% CI: -35.6 – -8.7) and in the BFR-PLA group by -14.8 ± 19.6% (95% CI: -27.9 – -1.6). Although the RMANOVA did not reveal significant interaction effects (p = .387, ηp² = 0.038), there was a significant main effect of time (p < 0.001, ηp² = 0.516). In our second acute protocol, regarding the exercise-induced ROS production during the last training session, ROS formation increased from pre- to post-exercise by 0.044 ± 0.169 µmol/l/min (95% CI: -0.069 – 0.158) and 0.066 ± 0.135 µmol/l/min (95% CI: -0.025 – 0.157) in the BFR-CH and BFR-PLA group, respectively. However, similar to the first training session, these results were not associated with a significant effect of time (p = 0.105, ηp² = 0.126) or interaction (p = 0.741, ηp² = 0.006) (Table 2).
Lifestyle parametersThe analysis of dietary behavior and physical activity were assessed before and after the intervention. For dietary intake, only the macronutrient intake was evaluated. Baseline data showed no significant difference between the groups (p > 0.05). No significant interaction effect was observed for fat (p = 0.327), protein (p = 0.103), carbohydrates (p = 0.266) or total calorie intake (p = 0.548). Regarding the level of regular physical activity, no statistically significant baseline differences were detected (p > 0.05). Additionally, there was no significant interaction effect (p = 0.548).
The current investigation sought to i) investigate the effects of BFR training on muscle mass and function in older men and ii) to evaluate the additional benefit of a daily dose of 15 g collagen hydrolysate. The main finding was that thigh CSA significantly increased following BFR training with and without CH ingestion. Although not statistically significant, percentage gain in muscle CSA after training was higher when CH was consumed compared to the BFR-PLA group (6.7% vs 5.7%). Muscular strength did not show significant adaptations between all groups. Studies consistently show low-load training with BFR to cause muscular hypertrophy (Vechin et al., 2015; Yasuda et al., 2014). However, evidence in older people is sparse. In terms of muscular hypertrophy, a study from Yasuda et al. (2014) reported that muscle CSA of thigh and hip muscles was increased by 4.4 – 8.0 % following 12-weeks of BFR training. The observed gains are in line with the data from our study. Comparing these changes to conventional high-load (HL) resistance training, Vechin et al. (2015) reported that both BFR and HL training are equally effective in increasing quadriceps CSA in a cohort of older men and women. Following 12-weeks of BFR and HL training, the participants’ muscle mass increased by 5.9% and 7.3%, respectively (Vechin et al., 2015). The results from our study show similar changes in only 8-weeks but with the same training volume. The responsible mechanisms for the hypertrophic effects of BFR training are not completely understood. Following an acute session of BFR exercise in older men, Fry et al. (Fry et al., 2010) reported an upregulation of mTOR signaling and muscle protein synthesis (+56%) with no changes in the control group. In general, this signaling pathway can be activated by various intracellular and extracellular inputs, such as energy status, oxygen availability, stress/load, growth factors or amino acids (Laplante and Sabatini, 2009; Marcotte et al., 2015). Older individuals, however, show reduced amino acid sensitivity and require higher protein intake compared to younger individuals (Baum et al., 2016). Recent evidence suggests that essential amino acids (especially leucin) are effective stimulators of muscle protein synthesis (Marcotte et al., 2015). Moreover, it has repeatedly been confirmed that arginine and glycine have also strong signaling properties regarding mTOR complex activation (2016; Yao et al., 2008). Collagen hydrolysate contains both arginine and glycine and might therefore have potential to influence anabolic processes. Interestingly, results from Kitakaze et al. (2016) have shown that dipeptides such as Hyp-Gly promote myogenic differentiation and hypertrophy in skeletal muscle cells by phosphorylating Akt, mTOR and S6K1 (Kitakaze et al., 2016). Considerable amounts of Hyp-Gly levels have been found in human blood following the ingestion of collagen hydrolysate (Shigemura et al., 2011). In addition to in vitro experiments, several previous investigations revealed that collagen peptides enhance the effects of resistance training regarding its influence on fat free mass and strength (Jendricke et al., 2019; Oertzen-Hagemann et al., 2019; Zdzieblik et al., 2015). Besides the crucial role of amino acids in this context, recent evidence indicates that both IGF-1 and ROS are likewise important second messengers leading to cellular adaptations and muscle remodulation (Powers et al., 2010). The results from our study show that the change in systemic serum IGF-1 concentrations does not significantly differ between the groups. While some studies state that circulating IGF-1 concentrations increase following BFR training, other trials have not found any changes (Manini et al., 2012). It must be noted that muscular hypertrophy is not always accompanied by an increased expression of systemic growth hormones following a 10-week resistance training program (Walker et al., 2004). In addition to growth hormone (GH) or IGF-1 secretion, it has been suggested that contraction-induced ROS are also involved in muscular remodulation (for review see (Powers et al., 2011)). The mitogen-activated protein kinase (MAPK) family, for example, is redox-sensitive and plays an important role in cellular adaptations and the regulation of several nuclear transcription factors (Powers et al., 2010). Studies investigating these effects in human skeletal muscle are scarce. Previous findings from our working group have demonstrated that ROS production is elevated following a single BFR session. Similarly, a study from Khoramipour et al. (2017) confirmed acute effects of BFR on enzymatic antioxidant activity (superoxide dismutase, glutathione peroxidase). Although there was no acute effect on ROS production in this trial, we demonstrated a significant chronic reduction of resting ROS generation following BFR training (both in BFR-CH and BFR-PLA groups). The present study is to our knowledge the first study to date that has combined BFR training with an additional protein supplementation. Although the results are not statistically significant, there is a net benefit of +1% in the BFR-CH group, compared to BFR-PLA group (6.7% vs. 5.7%). The non-significant finding might be due to generally small effect sizes of a post-exercise protein supplementation in untrained individuals (Morton et al., 2018), which demonstrates that further studies with bigger sample size are needed. As far as the second outcome, muscular strength, is concerned, no significant increases were observed. These findings are partly in line with the literature. Previous investigations confirm that low-load BFR training enhances strength when compared with low-load training with normal blood flow (Shinohara et al., 1998). A study from Vechin et al. (2015) found similar strength increases to our study by measuring leg press 1RM following 12 weeks of BFR training (~17%). Comparing these effects to HL training (~54%), significant differences were revealed. This can at least partly be explained by different changes in neural drive between BFR and HL training. In fact, studies show higher amplitudes in surface electromyography (EMG) during an acute HL training session compared to a BFR training session (Cook et al., 2013). Similar increases in EMG amplitude were reported for long-term HL training, with no significant changes in BFR resistance training (Kubo et al., 2006). In this study, moderate to large effect sizes for muscular strength were observed (ηp² = 0.098). However, despite the clinical relevance, these results did not reach statistical significance probably due to sample size.
PerspectivesNumerous investigations have demonstrated that the age-induced loss of muscle strength is accompanied by a decrease in postural control (Wolfson et al., 1995) and an increased incidence of falls (Moreland et al., 2004). A simultaneous decrease in muscle mass can additionally contribute to the development of various cardiovascular diseases in older age (Koopman and van Loon, 2009). In order to counteract the loss functional performance in older age Cruz-Jentoft and Landi (2014) recommend comprehensive and multidimensional approaches combining nutrition and physical exercise as main corner stones of sarcopenia interventions. Although several studies have shown that HL resistance training and protein supplementation (Zdzieblik et al., 2015) improve body composition and muscle strength in older adults, near-maximum loads are often difficult to implement into geriatric resistance training programs due to concomitant diseases and contraindications. The current results reveal that low-load BFR training enhances muscle CSA in older individuals. Regarding an additional protein supplementation, we observed a positive trend towards the BFR-CH group. However, these findings did not reach statistical significance due to the small sample size. Further research is needed to verify the observed effects in older populations.
The findings of the present study suggest that low-load blood flow restriction training is a potent training alternative for facilitating muscle hypertrophy in older men at risk of sarcopenia. The supplementation of collagen hydrolysate following BFR training showed a trend towards higher increases in thigh muscle cross-sectional area compared to the placebo group. These differences, however, did not reach statistical significance. Further studies with bigger sample sizes are required to further evaluate the potential benefit of an additional protein supplementation following BFR training.
ACKNOWLEDGEMENTS |
The authors would like to thank all participants who voluntarily participated in this study. Collagen hydrolysate was provided by the Collagen Research Institute (CRI), Kiel, Germany. However, CRI was not involved in the design, data collection or interpretation in this study. Additionally, authors have neither financial nor competing interest concerning the outcome of this investigation. The experiments comply with the current laws of the country in which they were performed. The authors declare no conflict of interest |
|
AUTHOR BIOGRAPHY |
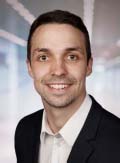 |
Christoph Centner |
Employment: Research Assistant at the Department of Sport and Sport Science, University of Freiburg, Germany |
Degree: MSc |
Research interests: Acute and chronic effects of blood flow restriction training on muscular adaptations |
E-mail: christoph.centner@sport.uni-freiburg.de |
|
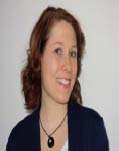 |
Denise Zdzieblik |
Employment: Research Assistant at the Department of Sport and Sport Science, University of Freiburg, Germany |
Degree: PhD |
Research interests: Effects of macro- and micronutrients on the functionality of the musculoskeletal system |
E-mail: denise.zdzieblik@sport.uni-freiburg |
|
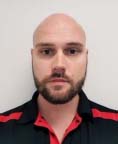 |
Llion Roberts |
Employment: Researcher & Lecturer in Human Physiology, School of Allied Health Sciences, Griffith University, Australia |
Degree: PhD |
Research interests: Integrated physiological responses to acute exercise and their associations and manipulations relating to training adaptations. |
E-mail: llion.roberts@griffith.edu.au |
|
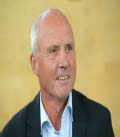 |
Albert Gollhofer |
Employment: Director of the Department of Sport and Sport Science, University of Freiburg, Germany |
Degree: Prof. Dr. |
Research interests: Neuromechanics and functional adaptation to training |
E-mail: albert.gollhofer@sport.uni-freiburg.de |
|
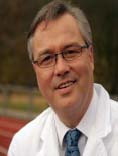 |
Daniel König |
Employment: Head of the Division of Nutrition at the Department of Sport and Sport Science, University of Freiburg, Germany |
Degree: Prof. Dr. med |
Research interests: Nutritional, preventive and sports medicine, lifestyle intervention, Nutrients and chronic disease |
E-mail: daniel.koenig@sport.uni-freiburg.de |
|
|
|
REFERENCES |
 Abellan van Kan G., Houles M., Vellas B. (2012) Identifying sarcopenia. Current Opinion in Clinical Nutrition & Metabolic Care 15, 436-441.
|
 ACSM (2009) American College of Sports Medicine position stand. Progression models in resistance training for healthy adults. Medicine & Science in Sports & Exercise 41, 687-708.
|
 Baar K., Esser K. (1999) Phosphorylation of p70(S6k) correlates with increased skeletal muscle mass following resistance exercise. American Journal of Physiology 276, C120-127.
|
 Baechle, T.R. and Earle, R.W. (2000) Essentials of Strength Training and
Conditioning. 2nd edition. Champaign, III: Human Kinetics.
|
 Baum J.I., Kim I.Y., Wolfe R.R. (2016) Protein Consumption and the Elderly: What Is the Optimal Level of Intake?. Nutrients 8, 359-367.
|
 Centner C., Zdzieblik D., Dressler P., Fink B., Gollhofer A., Konig D. (2018) Acute effects of blood flow restriction on exercise-induced free radical production in young and healthy subjects. Free Radical Research 52, 446-454.
|
 Cermak N.M., Res P.T., de Groot L.C., Saris W.H., van Loon L.J. (2012) Protein supplementation augments the adaptive response of skeletal muscle to resistance-type exercise training: a meta-analysis. The American Journal of Clinical Nutrition 96, 1454-1464.
|
 Cook S.B., Murphy B.G., Labarbera K.E. (2013) Neuromuscular function after a bout of low-load blood flow-restricted exercise. Medicine & Science in Sports & Exercise 45, 67-74.
|
 Cruz-Jentoft A.J., Baeyens J.P., Bauer J.M., Boirie Y., Cederholm T., Landi F., Martin F.C., Michel J.P., Rolland Y., Schneider S.M., Topinkova E., Vandewoude M., Zamboni M. (2010) Sarcopenia: European consensus on definition and diagnosis: Report of the European Working Group on Sarcopenia in Older People. Age Ageing 39, 412-423.
|
 Cruz-Jentoft A.J., Landi F. (2014) Sarcopenia. Clinical Medicine Journal 14, 183-186.
|
 English K.L., Paddon-Jones D. (2010) Protecting muscle mass and function in older adults during bed rest. Current Opinion in Clinical Nutrition & Metabolic Care 13, 34-39.
|
 Forbes G.B., Reina J.C. (1970) Adult lean body mass declines with age: some longitudinal observations. Metabolism 19, 653-663.
|
 Frey I., Berg A., Grathwohl D., Keul J. (1999) [Freiburg Questionnaire of physical activity--development, evaluation and application]. Sozial- und Präventivmedizin 44, 55-64.
|
 Fry C.S., Glynn E.L., Drummond M.J., Timmerman K.L., Fujita S., Abe T., Dhanani S., Volpi E., Rasmussen B.B. (2010) Blood flow restriction exercise stimulates mTORC1 signaling and muscle protein synthesis in older men. Journal of Applied Physiology (1985) 108, 1199-1209.
|
 Gheno R., Cepparo J.M., Rosca C.E., Cotten A. (2012) Musculoskeletal disorders in the elderly. Journal of Clinical Imaging Science 2, 39-.
|
 Heikkinen, E. (2008) What are the main risk factors for disability in old
age and how can disability be prevented? WHO Regional Office
for Europe, Copenhagen.
|
 Jendricke P., Centner C., Zdzieblik D., Gollhofer A., Konig D. (2019) Specific Collagen Peptides in Combination with Resistance Training Improve Body Composition and Regional Muscle Strength in Premenopausal Women: A Randomized Controlled Trial. Nutrients 11, 892.
|
 Khoramipour K., Dehghan P., Saboory M., Shahed A., Basereh A. (2017) Acute effects of submaximal cycling activity with blood flow restriction on superoxide dismutase and plasma glutathione peroxidase enzymes in healthy men: a pilot study. Medicina Dello Sport 70, 176-185.
|
 Kitakaze T., Sakamoto T., Kitano T., Inoue N., Sugihara F., Harada N., Yamaji R. (2016) The collagen derived dipeptide hydroxyprolyl-glycine promotes C2C12 myoblast differentiation and myotube hypertrophy. Biochemical and Biophysical Research Communications 478, 1292-1297.
|
 Koopman R., van Loon L.J. (2009) Aging, exercise, and muscle protein metabolism. Journal of Applied Physiology (1985) 106, 2040-2048.
|
 Kubo K., Komuro T., Ishiguro N., Tsunoda N., Sato Y., Ishii N., Kanehisa H., Fukunaga T. (2006) Effects of low-load resistance training with vascular occlusion on the mechanical properties of muscle and tendon. Journal of Applied Biomechanics 22, 112-119.
|
 Laplante M., Sabatini D.M. (2009) mTOR signaling at a glance. Journal of Cell Science 122, 3589-3594.
|
 Manini T.M., Yarrow J.F., Buford T.W., Clark B.C., Conover C.F., Borst S.E. (2012) Growth hormone responses to acute resistance exercise with vascular restriction in young and old men. Growth Hormone & IGF Research 22, 167-172.
|
 Manton K.G., Gu X. (2001) Changes in the prevalence of chronic disability in the United States black and nonblack population above age 65 from 1982 to 1999. Proceedings of the National Academy of Sciences of the United States of America 98, 6354-6359.
|
 Marcotte G.R., West D.W., Baar K. (2015) The molecular basis for load-induced skeletal muscle hypertrophy. Calcified Tissue International 96, 196-210.
|
 Mariappan N., Elks C.M., Fink B., Francis J. (2009) TNF-induced mitochondrial damage: a link between mitochondrial complex I activity and left ventricular dysfunction. Free Radical Biology and Medicine 46, 462-470.
|
 Moreland J.D., Richardson J.A., Goldsmith C.H., Clase C.M. (2004) Muscle weakness and falls in older adults: a systematic review and meta-analysis. Journal of the American Geriatrics Society 52, 1121-1129.
|
 Morton R.W., Murphy K.T., McKellar S.R., Schoenfeld B.J., Henselmans M., Helms E., Aragon A.A., Devries M.C., Banfield L., Krieger J.W., Phillips S.M. (2018) A systematic review, meta-analysis and meta-regression of the effect of protein supplementation on resistance training-induced gains in muscle mass and strength in healthy adults. British Journal of Sports Medicine 52, 376-384.
|
 Mrakic-Sposta S., Gussoni M., Montorsi M., Porcelli S., Vezzoli A. (2012) Assessment of a standardized ROS production profile in humans by electron paramagnetic resonance. Oxidative Medicine and Cellular Longevity 2012, 10.
|
 Oertzen-Hagemann V., Kirmse M., Eggers B., Pfeiffer K., Marcus K., de Marées M., Platen P. (2019) Effects of 12 Weeks of Hypertrophy Resistance Exercise Training Combined with Collagen Peptide Supplementation on the Skeletal Muscle Proteome in Recreationally Active Men. Nutrients 11, 1072.
|
 Powers S.K., Duarte J., Kavazis A.N., Talbert E.E. (2010) Reactive oxygen species are signalling molecules for skeletal muscle adaptation. Experimental Physiology 95, 1-9.
|
 Powers S.K., Ji L.L., Kavazis A.N., Jackson M.J. (2011) Reactive oxygen species: impact on skeletal muscle. Compr Physiol 1, 941-969.
|
 Sayer A.A., Robinson S.M., Patel H.P., Shavlakadze T., Cooper C., Grounds M.D. (2013) New horizons in the pathogenesis, diagnosis and management of sarcopenia. Age Ageing 42, 145-150.
|
 Schulz K.F., Altman D.G., Moher D. (2010) CONSORT 2010 statement: updated guidelines for reporting parallel group randomised trials. British Medical Journal (Clinical research ed.) 340, c332.
|
 Shigemura Y., Akaba S., Kawashima E., Park E.Y., Nakamura Y., Sato K. (2011) Identification of a novel food-derived collagen peptide, hydroxyprolyl-glycine, in human peripheral blood by pre-column derivatisation with phenyl isothiocyanate. Food Chemistry 129, 1019-1024.
|
 Shinohara M., Kouzaki M., Yoshihisa T., Fukunaga T. (1998) Efficacy of tourniquet ischemia for strength training with low resistance. European journal of applied physiology and occupational physiology 77, 189-191.
|
 Takano H., Morita T., Iida H., Asada K.-i., Kato M., Uno K., Hirose K., Matsumoto A., Takenaka K., Hirata Y., Eto F., Nagai R., Sato Y., Nakajima T. (2005) Hemodynamic and hormonal responses to a short-term low-intensity resistance exercise with the reduction of muscle blood flow. European Journal of Applied Physiology 95, 65-73.
|
 Tsekoura M., Kastrinis A., Katsoulaki M., Billis E., Gliatis J. (2017) Sarcopenia and Its Impact on Quality of Life. Advances in Experimental Medicine and Biology 987, 213-218.
|
 Vechin F.C., Libardi C.A., Conceicao M.S., Damas F.R., Lixandrao M.E., Berton R.P., Tricoli V.A., Roschel H.A., Cavaglieri C.R., Chacon-Mikahil M.P., Ugrinowitsch C. (2015) Comparisons between low-intensity resistance training with blood flow restriction and high-intensity resistance training on quadriceps muscle mass and strength in elderly. The Journal of Strength & Conditioning Research 29, 1071-1076.
|
 Walker K.S., Kambadur R., Sharma M., Smith H.K. (2004) Resistance training alters plasma myostatin but not IGF-1 in healthy men. Medicine & Science in Sports & Exercise 36, 787-793.
|
 Wolfson L., Judge J., Whipple R., King M. (1995) Strength is a major factor in balance, gait, and the occurrence of falls. The Journals of Gerontology. Series A, Biological sciences and Medical Sciences 50, 64-7.
|
 Yao K., Yin Y.L., Chu W., Liu Z., Deng D., Li T., Huang R., Zhang J., Tan B., Wang W., Wu G. (2008) Dietary arginine supplementation increases mTOR signaling activity in skeletal muscle of neonatal pigs. The Journal of Nutrition 138, 867-872.
|
 Yasuda T., Fukumura K., Fukuda T., Uchida Y., Iida H., Meguro M., Sato Y., Yamasoba T., Nakajima T. (2014) Muscle size and arterial stiffness after blood flow-restricted low-intensity resistance training in older adults. Scandinavian Journal of Medicine & Science in Sports 24, 799-806.
|
 Zdzieblik D., Oesser S., Baumstark M.W., Gollhofer A., König D. (2015) Collagen peptide supplementation in combination with resistance training improves body composition and increases muscle strength in elderly sarcopenic men: a randomised controlled trial. British Journal of Nutrition 114, 1237-1245.
|
|
|
|
|
|
|