|
|
|
ABSTRACT |
A side-to-side difference in the muscle size of the rectus abdominis has been suggested to increase the strain injury risk. Attenuating the difference in size of the rectus abdominis may decrease the injury risk. To explore ways to highly activate one side of the rectus abdominis, we aimed to clarify the activity levels of both sides of the muscle during asymmetric abdominal exercises. Fifteen male sprinters performed the following five asymmetric exercises for the right and left sides: (i) sit-up twist, (ii) oblique leg raise, (iii) side bridge, (iv) side bridge roll out with the elbow, and (v) side bridge roll out with the foot. Side bridge roll out with the elbow and that with the foot were performed using a wheeled platform. During the exercises, electromyographic signals were recorded bilaterally from the upper, central, and lower portions of the rectus abdominis. We calculated the root mean square of electromyograms during the concentric and eccentric phases of the exercises and normalized to that during maximal voluntary contractions. In all portions of the rectus abdominis, the root mean squares of electromyograms were significantly higher in the moving side than in the non-moving side during the concentric and eccentric phases of the side bridge, the side bridge roll out with the elbow and that with the foot (all p < 0.01), but not in sit-up twist or oblique leg raise. The root mean squares of electromyograms of all portions of the rectus abdominis in the moving side were significantly higher in the side bridge roll out with the elbow and that with the foot than in the side bridge during both phases (all p < 0.01). The results suggest that the application of the wheeled platform to side bridge is useful to highly activate one side of the rectus abdominis. |
Key words:
Surface electromyography, sit-up twist, oblique leg raise, side bridge, wheeled platform
|
Key
Points
- We examined the activity levels of both sides of the rectus abdominis during asymmetric abdominal exercises to explore ways to highly activate one side of the muscle.
- The activity levels of all portions of the rectus abdominis were significantly higher in the moving side than in the non-moving side during the concentric and eccentric phases of side bridge, and side bridges roll out using the wheeled platform, but not in sit-up twist or oblique leg raise.
- The activity levels of all portions of the rectus abdominis in the moving side were significantly higher in the side bridges roll out than in the side bridge during both phases
- These results suggest that the application of the wheeled platform to side bridge is a useful approach to highly activate one side of the rectus abdominis.
|
The rectus abdominis (RA) contributes to the generation of trunk flexion and lateral flexion torques (Knudson and Blackwell, 2000; Ikeda et al, 2009), and stability of the pelvis and lumbar spine positions (Lee, 2021). Side-to-side differences in RA size have been reported in athletes who engage in asymmetric sports, such as tennis (Sanchis-Moysi et al, 2010), golf (Izumoto et al, 2019), and long jump (Takahashi and Wakahara, 2019). For example, tennis players had a larger RA in the non-dominant side than in the dominant side, and the side-to-side difference in RA size was substantially larger in the lower portion (55%) than in the upper portion (18% [Sanchis-Moysi et al, 2010]). A case study (Connell et al, 2006) reported that all strain injuries in RA of tennis players (11/11 cases) occurred in its lower portion. Similarly, another study (Balius et al, 2012) also observed that most of the strain injuries in RA of tennis players (16/18 cases) occurred in its lower portion. These studies collectively suggest that side-to-side differences in the size of RA are associated with the risk of strain injury (Connell et al, 2006; Sanchis-Moysi et al, 2010; Balius et al, 2012). Thus, attenuating a side-to-side difference in RA size may help to decrease the risk of strain injury. A possible approach to attenuating the side-to-side difference in muscle size of RA is to perform asymmetric abdominal exercises (e.g., sit-up twist [SUT], oblique leg raise [OLR], and side bridge [SB]). SUT and OLR mainly include trunk flexion in addition to trunk rotation, indicating that both sides of RA are simultaneously activated. Hence, SUT and OLR may not be sufficient to induce a side-to-side difference in RA activity levels. Less information is available on the activity levels of both sides of RA during SUT and OLR. Meanwhile, SB involves lateral trunk flexion only to the lower side, and thus could selectively activate one side of RA. Indeed, a few studies have observed that the activity level of RA during SB tends to be higher in the lower side than in the upper side (García-Vaquero et al, 2012; McGill et al, 2013; Vaičienė et al, 2018). However, the magnitude of the side-to-side difference in activity level during SB was only approximately 10% of the maximal voluntary contraction (MVC), which may be insufficient to attenuate the side-to-side difference in RA size. A promising approach to highly activate one side of RA is the use of a wheeled platform. Escamilla et al. (2010) showed that a symmetric abdominal exercise with a wheeled platform (abdominal roll out [straight]) induced a higher RA activity level than traditional abdominal exercises (sit-up and crunch). This is attributed to the mechanical features of the wheeled platform, which can slide horizontally with minimal friction. If a wheeled platform is applied to SB, the activity level of one side of RA can be higher than that of a normal SB. However, this possibility has not been investigated. This study aimed to clarify the activity levels of both sides of RA during asymmetric abdominal exercises. We hypothesized that SB would display a larger side-to-side difference in the activity level of RA than SUT and OLR. It was also hypothesized that the activity level of RA in the lower side is higher in SB with the wheeled platform than in SB without the platform. The findings of the present study may provide a novel option for resistance exercises targeting unilateral RA.
Experimental approach to the problemTo test the above hypotheses, we selected the following five asymmetric exercises for the abdominal muscles (Figure 1): (i) SUT, (ii) OLR, (iii) SB, (iv) side bridge roll out with the elbow (SBE), and (v) side bridge roll out with the foot (SBF). After performing MVCs of trunk flexion and lateral trunk flexion, the subjects performed asymmetric abdominal exercises for the right and left sides. During the exercises, surface electromyography (EMG) signals were recorded bilaterally from the upper, central, and lower portions of RA (URA, CRA, and LRA, respectively) and from a portion of each of the external and internal obliques (EO and IO, respectively, Figure 2). The root mean square of EMG (RMS-EMG) was calculated for each concentric and eccentric phase of the exercises. The RMS-EMG of RA during the asymmetric abdominal exercises was normalized to that during MVC of trunk flexion, whereas that of EO and IO during the exercises was normalized to that during MVC of lateral flexion. Through these experimental procedures, we assessed the magnitude of side-to-side differences in abdominal muscle activities during asymmetric abdominal exercises.
SubjectsNineteen male collegiate sprinters (age: 20.3 ± 1.3 years, body mass: 65.4 ± 5.5 kg, height: 173.9 ± 5.3 cm, the personal best record for 100 m sprint: 11.30 ± 0.40 s, mean ± standard deviation [SD]) were recruited in this study for the following two reasons. First, sprinters have been reported to possess a larger RA size than non-athletes (Abe et al, 2000). Second, they present thinner skinfolds measured at eight sites, including the abdomen (Miller et al, 2021). The ages of the subjects ranged from 18 to 22 years. Fourteen sprinters specialized exclusively in sprinting events of 100–200 m and the remaining five sprinters specialized in sprinting event of 400 m. They had a minimum of three years of experience in sprint running, and none had injury to the lower back or lower extremity at the time of the experiment. The sample size was determined using a priori power analysis (G*Power version 3.1.9.7, Franz Faul, Universitat Kiel, Germany) with a power of 80%, an α error of 0.05, and an accepted effect size (partial η2) of 0.30. The effect size of 0.30 was chosen based on a previous study that showed a significant difference in the activity level of RA among different sit-up exercises (partial η2 = 0.31 [Sanchis-Moysi et al, 2015]). The results of power analysis indicated that the minimum sample size was 18. Thus, 19 subjects were recruited for this study. All subjects were fully informed of the purpose, procedures, and possible risks of this study, and provided written informed consent prior to their participation. This study was approved by the Ethics Committee of the authors’ institution (no. 21031) and conducted according to the principles of the Declaration of Helsinki.
ProceduresMVC
The subjects performed MVCs of trunk flexion and lateral trunk flexion as in previous studies (Escamilla et al, 2006; Silva et al, 2020). Before the MVC tests, the subjects performed a warm-up session with three submaximal contractions in each task. For MVC of trunk flexion, the subjects lay supine on the floor with the knee joints flexed at 90° (full extension = 0°) and the arms crossed over the chest. Two MVCs of trunk flexion were performed against manual resistance applied to their shoulders by an investigator. For MVC of lateral trunk flexion, the subjects lay lateral decubitus on the floor with the hip and knee joints fully extended, and the arms crossed over the chest. The subjects performed two MVCs of lateral trunk flexion to each of the right and left sides against manual resistance applied to their shoulder and hip of the upper side. During each MVC, the force was gradually increased to its maximum level over 1 to 2 s, and sustained over 2 to 3 s. The order of the MVCs and exercise sides was randomized across the subjects. A sufficient rest period of 2 min was allowed between the trials. At the end of the experiment, MVCs were repeated to evaluate the possible effects of muscular fatigue on EMG data.
Asymmetric exercisesAfter the initial MVC tests, the subjects performed a few repetitions of five asymmetric abdominal exercises (SUT, OLR, SB, SBE, and SBF) as a familiarization session under the supervision of a certified strength and conditioning specialist. Subsequently, two repetitions of the asymmetric abdominal exercises were performed in each direction or side as follows. In SUT (Figure 1a), the subjects lay supine on a mat with their knee joints flexed to 90° and their hands lightly touching the temples. Their feet were held tightly by the examiner. They raised and twisted their upper body until the right or left elbow touched the contralateral knee, and then returned to the initial position. In OLR (Figure 1b), the subjects lay supine on the mat and grasped the post of a power rack with their hands. In the initial position, the hip joints were flexed at 90°, and the knee joints were slightly flexed. They obliquely lowered their legs toward the right or left marker positioned 45° to the midline of the body. With their foot lightly touching the marker, they obliquely raised their legs and then returned to their initial positions. In SB (Figure 1c), the subjects lay on the right or left side with the knee and hip joints of the lower side fully extended. The knee and hip joints of the upper side were flexed at 90°. The elbow joint of the lower side was flexed at 90° was placed directly under the shoulder joint on the ipsilateral side. The hand of the upper side was positioned on the anterosuperior iliac spine (ASIS). In the initial position, the shoulder, hip, and ankle joints of the lower side were aligned in a straight line in the frontal plane. The subjects lowered their pelvis immediately before contact with the mat and then returned to the initial position. In SBE (Figure 1d) and SBF (Figure 1e), the initial position was the same as that of SB, except that the elbow (SBE) or foot (SBF) of the lower side was placed on a wheeled platform (SPIDER MACHO, Dreamteam Inc., Kanagawa, Japan). The subjects moved the wheeled platform craniocaudally as far as possible and then returned it to the initial position. In all asymmetric abdominal exercises, each of the concentric and eccentric phases was completed in 2 s with the aid of a metronome set to 60 bpm. The order of exercises, as well as the directions and sides of the exercises, was randomized across the subjects. A sufficient rest period of 2 min was provided between exercises. When they were unable to perform the exercises at the prescribed cadence or with a proper technique, an additional set was performed after a 2 min rest. Even after the familiarization session, two subjects could not perform SBE or SBF at the prescribed cadence, respectively. Thus, these subjects were required to perform an additional set of the exercise.
Data collection and analysisBipolar electrodes (DL-141, S&ME, Tokyo, Japan, inter-electrode distance: 12 mm) were placed on the right and left sides of the following five portions as in the study by Escamilla et al. (Escamilla et al, 2006; 2010): (i) URA, the center of the muscle belly in the upper portion of RA, and approximately 3 cm lateral and 5 cm superior to the center of the umbilicus; (ii) CRA, the center of the muscle belly in the central portion of RA, and approximately 3 cm lateral to the center of the umbilicus, and 5 cm inferior to URA; (iii) LRA, the center of the muscle belly in the lower portion of RA, and approximately 3 cm lateral to the center of the umbilicus, and 5 cm inferior to CRA; (iv) EO, the midpoint between the most inferior point of the costal margin of the ribs and ASIS; (v) IO, approximately 2 cm inferomedial to ASIS, within a triangle consisting of the inguinal ligament, lateral border of the rectus sheath and a line connecting ASISs. The border and fascicle orientation of each muscle (portion) were carefully identified using an ultrasonic apparatus (PROSOUND α7, Hitachi Aloka Medical, Japan). After careful preparation of the skin by shaving, abrading, and cleaning with alcohol, electrodes were placed parallel to the fascicle orientation of the corresponding muscles, and fixed with surgical tape. The ground electrode was attached to the right malleolus. The procedures of the above preparations were consistent with the SENIAM recommendation (Hermens et al, 2000). Raw EMG signals were pre-amplified and recorded on a computer at a sampling frequency of 1 kHz using an A/D converter (PowerLab 16SP, ADInstruments, Australia). The EMG signals were band-pass filtered between 5 and 500 Hz using computer software (LabChart ver.8, ADInstruments, Australia). The start of each MVC test was manually determined using an electrical signal from a synchronizer (PH-1250A-6SW, DKH, Japan, sampling frequency: 1 kHz). The RMS-EMG during MVC was calculated from 2.0 to 3.0 s after an offset of the electrical signal (a duration of 1.0 s). The average value of the two trials was used for normalization of RMS-EMG during the asymmetric abdominal exercises. RMS-EMGs during the exercises were calculated for each concentric and eccentric phase. To determine the start and end of the phases, the motion of the subjects was recorded with a digital video camera (EX-100, Casio, Japan) at 240 Hz in the sagittal plane for SUT and OLR, and in the frontal plane for SB, SBE, and SBF. The video was synchronized with the EMG data using an electrical signal from the synchronizer. The RMS-EMG values of the two repetitions were averaged for each exercise. These procedures of measuring RMS-EMG during resistance exercise were similar to our previous study (Kawama et al, 2021). During the asymmetric abdominal exercises, four subjects showed extremely high amplitudes of EMG in their abdominal muscles, possibly due to motion artifacts and/or noise. Thus, subsequent analyses were performed on the remaining 15 subjects. For URA, CRA, and LRA, the mean RMS-EMG values were normalized to those during MVC of trunk flexion performed before the asymmetric abdominal exercises. For EO and IO, the mean RMS-EMG values were normalized to those during MVC of the lateral trunk flexion of the ipsilateral side. The sides of the body were referred to as moving and non-moving sides according to the exercise direction or side. For example, the right side was defined as the moving side when the subjects were performing SUT and OLR in the right direction, and SB, SBE, and SBF on the right side. In these cases, the left side was the non-moving side. The ratio of the normalized RMS-EMG of the moving side to that of the non-moving side was calculated for each exercise as an index of the side-to-side difference in muscle activation.
Statistical analysisThe Shapiro-Wilk normality test was performed to assess the distribution of the normalized RMS-EMG data. The results showed that some data had a non-Gaussian distribution (p = 0.001 to 0.989). Therefore, the Wilcoxon signed-rank test was performed to compare RMS-EMGs between the moving and non-moving sides of the examined muscles during each exercise. The effect of exercise on RMS-EMG of the moving side was tested using the Friedman test followed by the Wilcoxon signed-rank test for the exercises that showed a significant side-to-side difference in RMS-EMG of all examined muscles (portions). The Friedman test was also used to determine the effect of exercise (SUT, OLR, SB, SBE, and SBF) on the ratio of RMS-EMG of the moving side to that of the non-moving side in each muscle (portion). When appropriate, the Wilcoxon signed-rank test was performed to identify a significant difference in the RMS-EMG ratios between exercises. In addition, the Wilcoxon signed-rank test was used to compare RMS-EMGs during MVC performed before and after asymmetric abdominal exercises. The level of statistical significance was set at p < 0.05. The significance level was adjusted for multiple tests using Bonferroni correction (i.e., α = 0.05/number of exercises or number of pairs of exercises). The effect size (r) and its 95% confidence interval (CI) were calculated for pairwise comparisons because some data of the present study had a non-Gaussian distribution. All statistical analyses were performed using a statistical software package (IBM SPSS Statistics, version 27.0, IBM Corporation, Armonk, USA) and a web application (Langtest, Atsushi Mizumoto, Kansai University, Japan [Mizumoto and Plonsky, 2016]).
RMS-EMGThe RMS-EMG during MVC of trunk flexion was signifycantly decreased after the asymmetric exercises in the left URA (p = 0.035, r = 0.38, 95% CI = -0.16 to 0.75) and the right LRA (p = 0.026, r = 0.41, 95% CI = -0.13 to 0.76). Meanwhile, there were no significant differences in RMS-EMG of the other muscles (portions) during MVC performed before and after the asymmetric exercises (p = 0.063 to 0.600, r = 0.10 to 0.34, 95% CI = -0.44 to 0.73). The RMS-EMGs of all portions of RA were significantly higher in the moving side than in the non-moving side during concentric phase of SB (all p < 0.001, r = 0.64 to 0.73, 95% CI: 0.20 to 0.87), SBE (all p < 0.001, r = 0.73, 95% CI: 0.35 to 0.91), and SBF (all p < 0.001, r = 0.73, 95% CI: 0.35 to 0.91, Figure 3). Meanwhile, there were no significant side-to-side differences in RMS-EMGs of any portions of RA during the concentric phase of SUT (p = 0.018 to 0.999, r = 0.01 to 0.43, 95% CI: -0.51 to 0.77) or OLR (p = 0.489 to 0.890, r = 0.03 to 0.13, 95% CI: -0.49 to 0.60). The RMS-EMGs of EO and IO were significantly higher in the moving side than in the non-moving side during the concentric phase of SB (all p < 0.001, r = 0.73, 95% CI: 0.35 to 0.91), SBE (all p < 0.001, r = 0.73, 95% CI: 0.35 to 0.91), and SBF (all p < 0.001, r = 0.73, 95% CI: 0.35 to 0.91). The RMS-EMG of IO was significantly higher in the moving side than in the non-moving side during the concentric phase of SUT (p < 0.001, r = 0.70, 95% CI: 0.26 to 0.89). In contrast, the RMS-EMG of EO was significantly higher in the non-moving side than in the moving side during the concentric phase of SUT (p = 0.004, r = 0.52, 95% CI: 0.01 to 0.82). The RMS-EMGs of all portions of RA were significantly higher in the moving side than in the non-moving side during eccentric phase of SB (p = 0.001 to 0.003, r = 0.54 to 0.64, 95% CI: 0.03 to 0.87), SBE (all p < 0.001, r = 0.73, 95% CI: 0.35 to 0.91), and SBF (all p < 0.001, r = 0.73, 95% CI: 0.35 to 0.91, Figure 4). Meanwhile, no significant differences were observed in RMS-EMGs of any portions of RA during the eccentric phase of SUT (p = 0.073 to 0.525, r = 0.12 to 0.33, 95% CI: -0.42 to 0.72) or OLR (p = 0.010 to 0.600, r = 0.10 to 0.47, 95% CI: -0.44 to 0.79). The RMS-EMGs of EO and IO were also significantly higher in the moving side than in the non-moving side during the eccentric phase of SB (all p < 0.001, r = 0.64 to 0.73, 95% CI: 0.20 to 0.91), SBE (all p < 0.001, r = 0.73, 95% CI: 0.35 to 0.91), and SBF (all p < 0.001, r = 0.73, 95% CI: 0.35 to 0.91). In contrast, the RMS-EMG of EO was significantly higher in the non-moving side than in the moving side during the eccentric phase of SUT (p = 0.003, r = 0.54, 95% CI: -0.03 to 0.82). Similarly, RMS-EMG of IO was significantly higher in the non-moving side than in the moving side during the eccentric phase of OLR (p = 0.006, r = 0.50, 95% CI: -0.02 to 0.80). The Friedman test showed a significant main effect of exercise (SB, SBE, and SBF) on RMS-EMGs of all portions of RA, EO, and IO in the moving side during the concentric phase (all p < 0.001). The RMS-EMGs of all portions of RA and IO in the moving side were significantly higher in SBE (p < 0.001, r = 0.68 to 0.73, 95% CI: 0.26 to 0.91) and SBF (p < 0.01, r = 0.51 to 0.70, 95% CI: -0.01 to 0.89) than in SB. Additionally, RMS-EMG of EO in the moving side was significantly higher in SBF (p = 0.01, r = 0.47, 95% CI: -0.01 to 0.79) than SB. Similarly, there was a significant main effect of exercise (SB, SBE, and SBF) on RMS-EMGs of all portions of RA, EO, and IO in the moving side during the eccentric phase (all p < 0.001). The RMS-EMGs of all portions of RA, EO, and IO in the moving side were significantly higher in SBE (all p < 0.01, r = 0.51 to 0.73, 95% CI: -0.01 to 0.91) and SBF (all p < 0.001, all r = 0.73, all 95% CI: 0.35 to 0.91) than in SB.
Ratio of RMS-EMG between moving and non-moving sidesThe Friedman test showed a significant main effect of exercise on the ratio of RMS-EMGs of all examined muscles between the moving and non-moving sides during the concentric phase (all p < 0.001, Figure 5). The RMS-EMG ratios of URA, CRA, LRA, and EO were significantly higher in SB (all p < 0.001, r = 0.70 to 0.73, 95% CI: 0.30 to 0.91), SBE (all p < 0.001, r = 0.70 to 0.73, 95% CI: 0.30 to 0.91), and SBF (all p < 0.001, r = 0.68 to 0.73, 95% CI: 0.30 to 0.91) than in SUT or OLR. The RMS-EMG ratio of IO was significantly higher in SB than in SUT, OLR, and SBF (all p < 0.001, r = 0.63 to 0.73, 95% CI: 0.17 to 0.91). Additionally, the RMS-EMG ratio of IO was significantly higher in SUT (p < 0.001, r = 0.73, 95% CI: 0.35 to 0.91), SBE (p < 0.001, r = 0.73, 95% CI: 0.35 to 0.91), and SBF (p < 0.001, r = 0.70, 95% CI: 0.30 to 0.89) than in OLR. The Friedman test revealed a significant main effect of exercise on the ratio of RMS-EMGs of all examined muscles between the moving and non-moving sides during the eccentric phase (all p < 0.001, Figure 6). The ratios of RMS-EMGs of all examined muscles were significantly higher in SB (p = 0.001 to 0.002, r = 0.58 to 0.73, 95% CI: 0.09 to 0.91), SBE (all p < 0.001, r = 0.73, 95% CI: 0.35 to 0.91), and SBF (all p < 0.001, r = 0.70 to 0.73, 95% CI: 0.30 to 0.91) than in SUT and OLR. The ratio of RMS-EMG of EO was significantly higher in OLR (p = 0.001, r = 0.59, 95% CI: 0.12 to 0.85) than in SUT.
The main finding of the present study was that RMS-EMGs of all portions of RA were higher in the moving side than in the non-moving side during the concentric and eccentric phases of SB, SBE, and SBF. Additionally, the ratios of RMS-EMGs in all portions of RA during both phases were higher in SB, SBE, and SBF than those in SUT and OLR. These findings support our first hypothesis that SB induces a larger side-to-side difference in RA activity level than SUT and OLR. Moreover, RMS-EMGs of all portions of RA in the moving side were found to be higher in SBE and SBF than in SB during both the concentric and eccentric phases. This finding supports our second hypothesis that SB with a wheeled platform requires a higher activity level of one side of RA than that without the device. These results suggest that SB is a resistance exercise that induce a greater side-to-side difference in RA activity level as compared to SUT and OLR, and that the application of the wheeled platform to SB is a useful approach to highly activate one side of RA. During the concentric and eccentric phases of SB, the activity levels of all portions of RA were higher in the moving side than in the non-moving side, whereas such side-to-side differences were not observed in SUT or OLR. This result partly corresponds to previous findings showing that the activity level of RA during SB tends to be higher in the moving side than in the non-moving side (García-Vaquero et al, 2012; McGill et al, 2003; Vaičienė et al, 2018). Additionally, the ratios of the activity levels in all portions of RA during both phases were higher in SB than in SUT and OLR. The results could be explained by a difference in the involvement of trunk flexion between SB and the other exercises (SUT and OLR). During SB, the subjects performed only lateral trunk flexion to the lower side, and little or no trunk flexion was required. Thus, preferential activation of the lower side of RA would be required to generate a lateral trunk flexion torque in SB. Meanwhile, the subjects mainly performed trunk flexion with some trunk rotations during SUT and OLR. In this case, simultaneous activation of both sides of RA would be required to generate trunk flexion torque. Therefore, the involvement of trunk flexion during exercises seems to be an important factor that affects the degree of side-to-side differences in RA activity levels. The activity levels of all portions of RA in the moving side were higher during the concentric and eccentric phases of SBE and SBF than during SB. The results of muscle activity levels could be interpreted with external forces and torques applied to the body. During SBE and SBF, the subjects moved the wheeled platform horizontally as far as possible. As the horizontal distance from the elbow to the foot increased, the external torque caused by the vertical ground reaction force should have increased and acted to flex the trunk upward. To maintain the posture against this external torque, all portions of RA on the lower side may be highly activated during SBE and SBF. In addition, we also have to take into account the horizontal component of the ground reaction force. The wheeled platform can easily move horizontally with minimal friction, indicating that no or minimal horizontal reaction force acted at the point of contact with the ground during SBE and SBF. In such a situation, an even higher level of activation may have been necessary for RA in the lower side to generate a large internal torque for lateral flexion and to move the platform in a controlled manner. In sports and rehabilitation, asymmetric abdominal exercises (SUT, OLR, and SB) may be prescribed to selectively activate one side of RA, EO, and IO. Our results demonstrated that the ratios of RMS-EMGs of all portions of RA, EO and IO were smaller in SUT and OLR than in SB. These results imply that SUT and OLR are insufficient to elicit a side-to-side difference in activity levels of RA, EO, and IO compared to SB. Furthermore, the activity levels of all portions of RA, EO, and IO of the moving side were higher in SBE and SBF than in SB during eccentric phase. These results suggest that use of wheeled platform during SB is a useful approach to highly activate the moving side of RA, EO, and IO. Although the wheeled platform is generally used in symmetric abdominal exercises (e.g., abdominal roll out with hands), the application of the platform in SB may be a novel option for resistance exercises targeting unilateral RA, EO, and IO. This study has some limitations that should be considered. First, the surface EMG is known to be susceptible to crosstalk from neighboring muscles. To reduce the effects of crosstalk, we carefully identified the belly and borderline of each muscle using ultrasonography. The subjects of this study consisted of male sprinters, who have larger abdominal muscles (RA, EO, and IO) than non-athletes (Abe et al, 2000). Moreover, our subjects had thinner subcutaneous adipose tissue in the abdomen (3.5 to 4.4 mm, data not shown) than young healthy men in a previous study (approximately 14.0 mm [Kanehisa et al, 2004]). Thus, crosstalk seems to be minimal, if any, in the present study. Second, there were decreases in RMS-EMGs of the left URA and right LRA during MVC after asymmetric abdominal exercises. To reduce the effects due to muscular fatigue, we randomized the order of exercises as well as the sides of the exercises across the subjects. Therefore, we assumed that muscular fatigue did not have a substantial influence on our main findings. Third, we only quantified the activity levels during the asymmetric abdominal exercises. Thus, it remains unclear whether the observed side-to-side difference in muscle activity level leads to a corresponding difference in muscle hypertrophy. A recent review indicated that the muscle activity level in one exercise session may not be a valid predictor of muscle hypertrophy (Vigotsky et al, 2022). This point will be the subject of future research. Finally, we focused on a limited population (male collegiate sprinters) to determine side-to-side differences in the activity levels of the abdominal muscles. Further studies should clarify the applicability of the findings to other populations.
The present study showed that RMS-EMGs of all portions of RA were higher in the moving side than in the non-moving side during the concentric and eccentric phases of SB, SBE, and SBF. Additionally, the ratios of RMS-EMGs in all portions of RA during both phases were higher in SB, SBE, and SBF than those in SUT and OLR. Moreover, RMS-EMGs of all portions of RA in the moving side were found to be higher in SBE and SBF than in SB during both the concentric and eccentric phases. These results suggest that SB is a resistance exercise that induce a greater side-to-side difference in RA activity level as compared to SUT and OLR, and that the application of the wheeled platform to SB is a useful approach to highly activate one side of RA. The SB, SBE, and SBF may be helpful in decreasing the side-to-side difference in the muscle size of RA.
ACKNOWLEDGEMENTS |
The authors would like to thank everyone who contributed to the present study. The experiments complied with the current laws of the country in which they were performed. The authors have no conflicts of interest to declare. The datasets generated and analyzed during the current study are not publicly available, but are available from the corresponding author who was an organizer of the study. |
|
AUTHOR BIOGRAPHY |
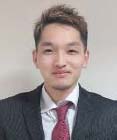 |
Raki Kawama |
Employment: Graduate School of Health and Sports Science, Doshisha University, Kyoto, Japan. Research Fellow of Japan Society for the Promotion of Science, Tokyo, Japan |
Degree: MSc, PhD student, NSCA-CSCS |
Research interests: Skeletal muscle, resistance exercise, injury prevention |
E-mail: rkawama156413@gmail.com |
|
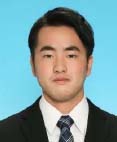 |
Akira Ike |
Employment: Faculty of Health and Sports Science, Doshisha University, Kyoto, Japan |
Degree: BSc student |
Research interests: Skeletal muscle, resistance exercise |
E-mail: gtd0051@mail4.doshisha.ac.jp |
|
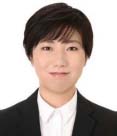 |
Ai Soma |
Employment: Faculty of Health and Sports Science, Doshisha University, Kyoto, Japan |
Degree: BSc student |
Research interests: Skeletal muscle, resistance exercise |
E-mail: gtd0193@mail4.doshisha.ac.jp |
|
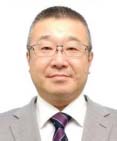 |
Tatsuya Hojo |
Employment: Faculty of Health and Sports Science, Doshisha University, Kyoto, Japan |
Degree: PhD, MD |
Research interests: Sports injury prevention, musculoskeletal rehabilitation |
E-mail: thojo@mail.doshisha.ac.jp |
|
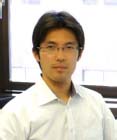 |
Taku Wakahara |
Employment: Faculty of Health and Sports Science, Doshisha University, Kyoto, Japan |
Degree: PhD |
Research interests: Skeletal muscle mechanics, resistance training |
E-mail: twakahar@mail.doshisha.ac.jp |
|
|
|
REFERENCES |
 Abe T., Kumagai K., Brechue W.F. (2000) Fascicle length of leg muscles is greater in sprinters than distance runners. Medicine & Science in Sports & Exercise 32, 1125-1129. Crossref
|
 Balius R., Pedret C., Galilea P., Idoate F., Ruiz-Cotorro A. (2012) Ultrasound assessment of asymmetric hypertrophy of the rectus abdominis muscle and prevalence of associated injury in professional tennis players. Skeletal Radiology 41, 1575-1581. Crossref
|
 Connell D., Ali K., Javid M., Bell P., Batt M., Kemp S. (2006) Sonography and MRI of rectus abdominis muscle strain in elite tennis players. American Journal of Roentgenology 187, 1457-1461. Crossref
|
 Escamilla R.F., Babb E., DeWitt R., Jew P., Kelleher P., Burnham T., Busch J., D’Anna K., Mowbray R., Imamura R.T. (2006) Electromyographic analysis of traditional and nontraditional abdominal exercises: implications for rehabilitation and training. Physical Therapy 86, 656-671. Crossref
|
 Escamilla R.F., Lewis C., Bell D., Bramblet G., Daffron J., Lambert S., Pecson A., Imamura R., Paulos L., Andrews J.R. (2010) Core muscle activation during Swiss ball and traditional abdominal exercises. Journal of Orthopaedic & Sports Physical Therapy 40, 265-276. Crossref
|
 García-Vaquero M.P., Moreside J.M., Brontons-Gil E., Peco-González N., Vera-Garcia F.J. (2012) Trunk muscle activation during stabilization exercises with single and double leg support. Journal of Electromyography and Kinesiology 22, 398-406. Crossref
|
 Hermens HJ, Freriks B, Disselhorst-Klug C., Rau G. (2000) Development of recommendations for SEMG sensors and sensor placement procedures. Journal of Electromyography and Kinesiology 10, 361-374. Crossref
|
 Ikeda Y., Miyatsuji K., Kawabata K., Fuchimoto T., Ito A. (2009) Analysis of trunk muscle activity in the side medicine-ball throw. The Journal of Strength & Conditioning Research 23, 2231-2240. Crossref
|
 Izumoto Y., Kurihara T., Suga T., Isaka T. (2019) Bilateral differences in the trunk muscle volume of skilled golfers. Plos One 14, e0214752. Crossref
|
 Kanehisa H., Miyatani M., Azuma K., Kuno S., Fukunaga T. (2004) Influences of age and sex on abdominal muscle and subcutaneous fat thickness. European Journal of Applied Physiology 91, 534-537. Crossref
|
 Kawama R, Takahashi K, Wakahara T (2021) Effect of Hip Joint Position on Electromyographic Activity of the Individual Hamstring Muscles During Stiff-Leg Deadlift. The Journal of Strength & Conditioning Research 35, 38-43. Crossref
|
 Knudson D., Blackwell J. (2000) Trunk muscle activation in open stance and square stance tennis forehands. International Journal of Sports Medicine 21, 321-324. Crossref
|
 Lee K. (2021) The Relationship of Trunk Muscle Activation and Core Stability: A Biomechanical Analysis of Pilates-Based Stabilization Exercise. International Journal of Environmental Research and Public Health 18, 12804. Crossref
|
 McGill S.M., Grenier S., Kavcic N., Cholewicki J. (2003) Coordination of muscle activity to assure stability of the lumbar spine. Journal of Electromyography and Kinesiology 13, 353-359. Crossref
|
 Miller R., Balshaw T.G., Massey G.J., Maeo S., Lanza M.B., Johnston M., Allen S.J., Folland J.P. (2021) The Muscle Morphology of Elite Sprint Running. Medicine & Science in Sports & Exercise 53, 804-815. Crossref
|
 Mizumoto A., Plonsky L.R. (2016) R as a lingua franca: Advantages of using R for quantitative research in applied linguistics. Applied Linguistics 37, 284-291. Crossref
|
 Sanchis-Moysi J., Idoate F., Dorado C., Alayón S., Calbet J.A.L. (2010) Large asymmetric hypertrophy of rectus abdominis muscle in professional tennis players. Plos One 5, e15858. Crossref
|
 Silva F.H.O., Arantes F.J., Gregorio F.C., Santos F.R.A., Fidale T.M., Bérzin F., Bigaton D.R., Lizardo F.B. (2020) Comparison of the Electromyographic Activity of the Trunk and Rectus Femoris Muscles During Traditional Crunch and Exercise Using the 5-Minute Shaper Device. Journal of Strength & Conditioning Research 34, 1-10. Crossref
|
 Sullivan W., Gardin F.A., Bellon C.R., Leigh S. (2015) Effect of Traditional vs. Modified Bent-Knee Sit-Up on Abdominal and Hip Flexor Muscle Electromyographic Activity. Journal of Strength & Conditioning Research 29, 3472-3479. Crossref
|
 Takahashi K., Wakahara T. (2019) Association between trunk and gluteus muscle size and long jump performance. Plos One 14, e0225413. Crossref
|
 Vaičienė G., Berškienė K., Slapsinskaite A., Mauricienė V., Razon S. (2018) Not only static: Stabilization Manoeuvres in Dynamic Exercises - A pilot study. Plos One 13, e0201017. Crossref
|
 Vigotsky A.D., Halperin I., Trajano G.S., Vieira TM (2022) Longing for a Longitudinal Proxy: Acutely Measured Surface EMG Amplitude is not a Validated Predictor of Muscle Hypertrophy. Sports Medicine 52, 193-199. Crossref
|
|
|
|
|
|
|