|
|
|
ABSTRACT |
Dynamic stretching for more than 90 seconds is useful for improving muscle strength, although dynamic stretching for 30 seconds or less is commonly used in sports settings. The effects of dynamic stretching are influenced by the speed and amplitude of stretching, but no study examined these factors for 30 seconds of dynamic stretching. Therefore, the purpose of the present study was to examine the effects of speed (fast- or slow-speed) and amplitude (normal- or wide amplitude) of dynamic stretching for 30 seconds on the strength (peak torque during maximum isokinetic concentric contraction) and flexibility (range of motion, passive torque at maximum knee extension angle, and muscle-tendon unit stiffness) of the hamstrings. The passive torque and muscle-tendon unit stiffness reflect stretching tolerance and viscoelastic properties of the hamstrings, respectively. Fifteen healthy participants performed 4 types of 30 seconds of dynamic stretching. The muscle strength and flexibility were measured before and immediately after the dynamic stretching. The range of motion did not change after dynamic stretching at low speed and normal amplitude (p = 0.12, d = 0.59, 103.3%), but it was increased by other interventions (p < 0.01, d = 0.90-1.25, 104.5-110.1%). In all interventions, the passive torque increased (main effect for time, p < 0.01, d = 0.51 – 0.74, 111.0 – 126.9%), and muscle-tendon unit stiffness did not change. The muscle strength increased only after dynamic stretching at fast speed with normal amplitude (p < 0.01, d = 0.79, 107.1%). The results of the present study indicated that 30 seconds of dynamic stretching at fast speed and with normal amplitude can be beneficial for the measured parameters. |
Key words:
Range of motion, peak torque, passive torque, stiffness, warm-up routine, short duration
|
Key
Points
- We investigated the acute effects of different speeds and intensities of dynamic stretching on strength and flexibility of the hamstrings
- In all interventions, the passive torque increased, and muscle-tendon unit stiffness did not change.
- The muscle strength of the hamstrings increased only after dynamic stretching at fast speed (60rpm) with normal amplitude (within range of motion).
|
Dynamic stretching is part of a warm-up program before sports competitions to improve muscle performance and flexibility and prevent injuries (Takeuchi et al., 2019; Judge et al., 2020). Dynamic stretching is performed by contracting antagonist muscles at a controlled tempo within the range of motion (ROM) of the joint (Bandy et al., 1998; Jaggers et al., 2008; Samukawa et al., 2011; Matsuo et al., 2019; Iwata et al., 2019). The effect of dynamic stretching on muscle strength is influenced by the duration of stretching (Behm and Chaouachi, 2011). It was demonstrated that dynamic stretching for a longer duration (more than 90 seconds) increased several performances to a great extent (muscle strength, power, jump, etc.) compared to dynamic stretching for a shorter duration (less than 90 seconds) (Behm and Chaouachi, 2011). However, in a sports setting, a recent survey study reported that dynamic stretching for 30 seconds or less was commonly used as a part of the warm-up routine to improve muscle performance (Takeuchi et al., 2019). For many athletes, the duration of sports practice is very limited. Therefore, it is necessary to develop a dynamic stretching method that can effectively improve muscle strength within 30 seconds. Some previous studies showed significant improvement in muscle performance after a short duration of dynamic stretching (Yamaguchi and Ishii, 2005; Yamaguchi et al., 2007; Fletcher, 2010; Behm et al., 2011). However, these previous studies performed dynamic stretching on multiple muscles (total of more than 90 seconds of stretching) (Yamaguchi and Ishii, 2005; Yamaguchi et al., 2007; Fletcher, 2010; Behm et al., 2011), and it is unclear whether this can be considered an effect of short-duration dynamic stretching. Moreover, these previous studies did not describe the speed and amplitude of the dynamic stretching. Dynamic stretching at fast speeds is useful for improving muscle performance compared to low speeds due to an increment in heart rate (Fletcher and Anness, 2007). On the other hand, dynamic stretching at uncontrolled speeds and amplitude inhibits the improvement of muscle performance after the stretch (Bandy et al., 1997). In addition, fatigue of dynamic stretching inhibits the improvement of muscle strength after dynamic stretching (Mizuno, 2022). Therefore, in order to develop an effective dynamic stretching program as a part of a warm-up program, it is necessary to examine in detail the effective speed and amplitude of 30 seconds of dynamic stretching for improving muscle strength. To our best knowledge, there is no study examining the effects of dynamic stretching for a short duration on a change in flexibility (ROM and muscle-tendon unit stiffness), which is related to the occurrence of muscle tendon injuries (Kaufman et al., 1999; Watsford et al., 2010; Pickering et al., 2017). Changes in ROM after stretching are attributed to changes in stretching tolerance and viscoelastic properties of the muscle. The stretching tolerance and viscoelastic properties are measured by using passive torque at maximum ROM and muscle-tendon unit stiffness. Therefore, the purpose of the present study was to examine the effects of speed (fast- or slow-speed) and amplitude (normal- or wide-amplitude) of dynamic stretching on the strength and flexibility of the hamstrings. It was hypothesized that dynamic stretching performed at fast speed and normal amplitude would be useful for increasing the strength of the hamstrings, based on previous studies (Bandy et al., 1997; Fletcher and Anness, 2007).
Experimental approach to the problemA randomized repeated-measures experimental design was used to examine the effects of speed and amplitude of dynamic stretching on flexibility and strength of the hamstrings. The participants underwent four different interventions (Slow-Normal, Slow-Wide, Fast-Normal, and Fast-Wide) of dynamic stretching with an interval of ≧ 24 hours between visits, in random order (Figure 1). Participants attended a familiarization session 1 week before the first testing day. In the familiarization session, participants experienced the speed and amplitude of each dynamic stretching intervention, and muscle strength and flexibility measurements. The data collection was as follows: rest for 5 minutes (no warm-up), pre flexibility measurement, pre muscle strength measurement, dynamic stretching intervention, post flexibility measurement, and post muscle strength measurement. To assess changes in the flexibility of the hamstrings in the dominant limb (ball kicking preference) (Takeuchi et al, 2021b), ROM, passive torque at maximum knee extension angle, and muscle-tendon unit stiffness were measured before and immediately after dynamic stretching. After the flexibility measurement, the peak torque during maximum concentric contraction was measured to evaluate the muscle strength of the hamstrings. In addition, heart rate and leg fatigue during stretching were assessed. The experiment was performed in a university laboratory, where the temperature was maintained at 25 °C.
ParticipantsNine healthy recreationally active men (20.8 ± 0.3 years, 1.74 ± 0.05 m, 64.8 ± 10.0 kg) and six healthy women (20.7 ± 0.4 years, 1.60 ± 0.05 m, 52.0 ± 6.6 kg) were recruited. The inclusion criteria were that participants did not regularly perform any flexibility and strength training, and those who had no history of lower limb pathology. The sample size of the muscle-tendon unit stiffness was calculated with a power of 80%, alpha error of 0.05, and effect size f of 0.25 (middle) (Fletcher, 2010) using G*Power 3.1 software (Heinrich Heine University, Düsseldorf, Germany), and the results showed that the requisite number of participants for this study was 12 participants; thus, 15 participants were recruited to account for possible attrition. All participants were informed of the requirements and risks associated with their involvement in this study and signed a written informed consent document. The study was performed in accordance with the Declaration of Helsinki (1964). The Ethics Committee of Kobe International University approved the study (No. G2022-170).
Sitting position of measurementsThe flexibility assessment was performed in the same fashion as previous studies (Takeuchi and Nakamura, 2020b; Takeuchi et al., 2021a; 2021b; 2021c). An isokinetic dynamometer machine (CYBEX NORM, Humac, California, USA) was used in the present study. This study used a sitting position with the hip joint flexed, which has been shown to efficiently stretch the hamstrings (Kataura et al., 2017). The participants were seated on a chair with the seat tilted maximally, and a wedge-shaped wooden flame was inserted between the trunk and the backrest, which set the angle between the seat and the back at approximately 60°. A previous study, which used the same assessment, eported that the average angle of hip flexion was 111.2° ± 2.5° (Kataura et al., 2017). The chest, pelvis, and right thigh were stabilized with straps. The right knee joint was aligned with the axis of the rotation of the isokinetic dynamometer machine. The lever arm attachment was placed just proximal to the malleolus medialis and stabilized with straps. In the present study, reported knee angles were measured using the isokinetic dynamometer machine. A 90° angle between the lever arm and floor was defined as 0° of knee flexion/extension. The participants were instructed to relax during the flexibility assessment.
Range of motion, passive torque, and muscle-tendon unit stiffnessROM and passive torque were measured by using the isokinetic dynamometer. The knee joint was passively extended from 0 degrees to the maximum angle without pain at 5 degrees/second. A previous study showed that this velocity does not cause a stretch reflex (Morse, 2011). ROM was defined as the range from 0 degrees to the maximum knee extension angle. The passive torque during ROM measurement was recorded in the isokinetic dynamometer. After the experiment, the knee extension angle and passive torque during the flexibility measurement were exported to a personal computer, and the passive torque and muscle-tendon unit stiffness were analyzed. The passive torque at the maximum knee extension angle was used for further analysis. The muscle-tendon unit stiffness of the hamstrings was defined as the values of the slope of the regression line that was calculated from the torque-angle curve using the least-squares method (Magnusson et al., 1997; Kataura et al., 2017; Takeuchi and Nakamura, 2020b; Takeuchi et al., 2021a). The muscle-tendon unit stiffness was calculated from the same knee extension angle range before and after dynamic stretching. The calculated knee extension angle range was defined as the angle from the 50% maximum knee extension angle to the maximum knee extension angle measured before dynamic stretching (Kataura et al., 2017; Takeuchi and Nakamura, 2020b; Takeuchi et al., 2021b). However, if the maximum knee extension angle measured after static stretching was smaller than that before stretching, the muscle-tendon unit stiffness was calculated from the 50% maximum knee extension angle to the maximum knee extension angle measured after stretching (Kataura et al., 2017; Takeuchi and Nakamura, 2020b; Takeuchi et al., 2021b).
Muscle strengthThe muscle strength assessment was performed in the same fashion as a previous study (Takeuchi and Nakamura, 2020a). The peak torque of knee flexion during maximum voluntary isokinetic concentric contraction at 60 degrees/second was measured. The participants were secured on the isokinetic dynamometer machine in the same fashion as the flexibility assessment. The range of movement was set from 0 degrees to maximum knee extension angle. The participants performed three submaximal trials on the isokinetic dynamometer machine. After the submaximal trials, the participants performed three maximum voluntary isokinetic concentric contractions. The greatest value of the three repetitions was used for the analyses.
Dynamic stretchingParticipants performed dynamic stretching of the dominant leg for 30 seconds under four different conditions. No warm-up was performed to eliminate the potential interaction between warm-up and dynamic stretching. Participants stood upright with each hand grasping a horizontal bar at waist height. Then, participants flexed their hip joint with their knee joint extended at a tempo dictated by a metronome so that their dominant leg swung up to their anterior aspect and their hamstrings were stretched. In Slow-Normal and Fast-Normal, dynamic stretching was performed at the hip flexion range just before any pain in the hamstrings, the range which was confirmed before the stretching intervention by slowly flexing the hip joint to the maximum angle. In Slow-Wide and Fast-Wide, dynamic stretching was performed at the maximum hip flexion angle which could maintain the tempo of stretching without any compensations. The amplitude of each dynamic stretching is shown in the results section. During dynamic stretching, two examiners confirmed that no trunk or knee joint compensations occurred. To measure the amplitude of dynamic stretching, reflective markers were placed on the greater trochanter and lateral malleolus (Takeuchi et al., 2021c). A video camera was used to capture an image of the sagittal plane during stretching. The range of the hip joint of all leg swings was measured by using Image J software (National Institutes of Health). A line perpendicular to the floor was defined as 0 degrees of hip flexion/extension, and the angle between the perpendicular to the floor and the line passing through the greater trochanter and lateral malleolus was measured. The total angle of the hip joint during dynamic stretching was calculated from hip flexion and extension range per leg swing. In addition, the angular velocity (degree/second) of dynamic stretching was calculated from the total angle (degree) and tempo (second per leg swing) of dynamic stretching.
Heart rate and leg fatigueHeart rate was assessed before and immediately after dynamic stretching by using wearable wireless sensors (PULSEENSE, SEIKO, Japan). The level of subjective fatigue of dynamic stretching was quantified by an 11-point numerical rating scale (NRS) that ranged from 0 (feel no fatigue) to 10 (feeling too fatigued to leg swing anymore).
ReliabilityThe test-retest reliability for all dependent variables was determined in 4 men and 4 women. The 2 tests were separated by 3 days and were performed at the same time of the day. The reliability of knee extension ROM (intraclass correlation coefficient (ICC) of 0.96 and 95% confidence interval (95%CI) of 0.84-0.99), passive torque at the maximum knee extension angle (ICC of 0.89 and 95%CI of 0.61-0.97), muscle-tendon unit stiffness (ICC of 0.93 and 95%CI of 0.76-0.98), and muscle strength (ICC of 0.89 and 95%CI of 0.61-0.97) were acceptable in this study.
Statistical analysisStatistical analysis was performed according to previous studies (Takeuchi et al., 2021e). The distribution of the data was assessed using the Shapiro–Wilk test, and it was confirmed that the data followed a normal distribution. All variables were described as mean ± standard deviation. A one-way repeated ANOVA was used to analyze amplitude (flexion, extension, and total range) and angular velocity of dynamic stretching and NRS data. For heart rate, ROM, passive torque, muscle-tendon unit stiffness, and muscle strength data, a two-way repeated ANOVA (time [pre vs. post] and conditions [Slow-Normal vs. Slow-Wide vs. Fast-Normal vs. Fast-Wide]) was used to analyze the interaction and main effect. If a significance was detected, post hoc analyses using Bonferroni’s test were performed to determine where significant differences occurred. Partial eta squared values were reported to reflect the magnitude of the differences for each treatment (small = 0.01, medium = 0.06, and large = 0.14) (Cohen, 1988). Cohen’s d was calculated as the mean difference between pre and post values, divided by the pooled standard deviation between pre and post (Cohen, 1988). A d of 0.00–0.19 was considered trivial, 0.20–0.49 was considered small, 0.50–0.79 was considered moderate, and ≥ 0.80 was considered large. The analyses were performed using SPSS version 25 (SPSS, Inc., Chicago, IL, USA). Differences were considered statistically significant at an alpha level of p < 0.05.
Range of motion, passive torque, and muscle-tendon unit stiffnessFor ROM, there was a significant interaction (p = 0.03, partial eta squared = 0.19) (Figure 2). ROM in Slow-Wide (p < 0.01, d = 0.90), Fast-Normal (p < 0.01, d = 0.92), and Fast-Wide (p < 0.01, d = 1.25) increased, but it was not changed in Slow-Normal (p = 0.12, d = 0.59). For the passive torque, there was no significant interaction (p = 0.87, partial eta squared = 0.02) and no main effect for intervention (p = 0.30, partial eta squared = 0.08), but there was a significant main effect for time (p < 0.01, partial eta squared = 0.71) (Figure 2). Passive torque increased after stretching interventions (Slow-Normal, d = 0.51; Slow-Wide, d =0.72; Fast-Normal, d= 0.74; Wide-Normal; d = 0.59). For the muscle-tendon unit stiffness, there was no significant interaction (p = 0.18, partial eta squared = 0.11), no main effect for intervention (p = 0.74, partial eta squared = 0.03) or time (p = 0.61, partial eta squared = 0.02) (Figure 2).
Muscle strengthFor muscle strength, there was a significant interaction (p = 0.03, partial eta squared = 0.19) (Figure 3). Muscle strength increased in Fast-Normal (from 52.8 ± 20.6 Nm to 55.3 ± 20.4 Nm, p < 0.01, d = 0.79), but it was not changed in Slow-Normal (from 53.7 ± 19.1 Nm to 52.8 ± 17.3 Nm, p = 0.44, d = 0.34), Slow-Wide (from 51.1 ± 20.1 Nm to 51.8 ± 19.7 Nm, p = 0.92, d = 0.02), or Fast-Wide (from 52.9 ± 23.0 Nm to 52.6 ± 23.1 Nm, p = 0.78, d = 0.07).
Heart rate and NRSFor heart rate, there was no significant interaction (p = 0.30, partial eta squared = 0.08) and no main effect for intervention (p = 0.21, partial eta squared = 0.10), but there was a significant main effect for time (p < 0.01, partial eta squared = 0.66). Heart rate significantly increased after the stretching intervention (Slow-Normal, from 65.9 ± 3.7 bpm to 78.9 ± 15.3 bpm, d = 0.94; Slow-Wide, from 69.5 ± 9.2 bpm to 79.6 ± 9.2 bpm, d = 0.71; Fast-Normal, from 69.2 ± 8.7 bpm to 85.7 ± 25.1 bpm, d = 0.73; Fast-Wide, from 68.9 ± 7.7 bpm to 91.7 ± 26.2 bpm, d = 0.87) (p < 0.01). For NRS, there was a significant interaction (p < 0.01, partial eta squared = 0.34). Slow-Normal (2.1 ± 0.8) was significantly lower than Fast-Normal (3.0 ± 0.9) (p = 0.03) and Fast-Wide (p = 0.02). There was no significant difference between Slow-Normal and Slow-Wide (2.5 ± 1.0) (p = 0.49). There was no significant difference between Slow-Wide, Fast-Normal, and Fast-Wide (3.5 ± 1.2) (Slow-Wide and Fast-Normal, p = 0.72; Slow-Wide and Fast-Wide, p = 0.26; Fast-Normal and Fast-Wide, p = 0.67).
Amplitude and angular velocity of dynamic stretchingFor the amplitude of dynamic stretching, there was a significant interaction in the hip flexion (p < 0.01, partial eta squared = 0.54), extension (p < 0.01, partial eta squared = 0.46), and total ranges (p < 0.01, partial eta squared = 0.60) of dynamic stretching (Table 1). In hip flexion and total ranges, Slow-Wide was significantly higher than Slow-Normal (p < 0.05) and Fast-Normal (p < 0.01). In all amplitudes, Fast-Wide was significantly higher than Slow-Normal (p < 0.01), Slow-Wide (p < 0.01), and Fast-Normal (p < 0.01). For the angular velocity of dynamic stretching, there was a significant interaction (p < 0.01, partial eta squared = 0.90). Slow-Normal was significantly lower than Slow-Wide (p = 0.03), Fast-Normal (p < 0.01), and Fast-Wide (p < 0.01). Slow-Wide was significantly lower than Fast-Normal (p < 0.01) and Fast-Wide (p < 0.01). Fast-Normal was significantly lower than Fast-Wide (p < 0.01).
Although many previous studies examined effective techniques of dynamic stretching for improving muscle strength, none have studied the detailed conditions (speed and amplitude) of 30 seconds of dynamic stretching, which is commonly used in sports settings. The present study compared the effects of 4 different speeds and amplitudes of dynamic stretching for 30 seconds on the muscle strength and flexibility of the hamstrings. The results of the study showed that dynamic stretching for 30 seconds at a fast speed and normal amplitude (within ROM) was useful for improving the muscle strength of the hamstrings. Muscle strength increased only in the Fast-Normal dynamic stretching protocol. Fletcher compared fast (100 bpm) and low speed (50 bpm) dynamic stretching consisting of 100 repetitions (10 repetitions × 2 sets × 5 exercises) and showed that fast speed dynamic stretching was better for improving jump performance than slow speed because of an increment in heart rate (Fletcher, 2010). In the present study, an increment in heart rate after dynamic stretching would not be related to increment in the muscle strength in Fast-Normal because all interventions increased the heart rate without any difference of change. Post-activation potentiation enhancement (PAPE) is one of the important mechanisms for increment in muscle strength after dynamic stretching (Opplert and Babault, 2018). PAPE is a transient improvement of muscle contractile performance after previous contractile activities (Sale, 2002). The effect of PAPE is related to the degree of muscular recruitment of the contraction (Sale, 2002; Hough et al., 2009; Opplert and Babault, 2018), and fast speed dynamic stretching increases EMG amplitude compared to low speed (Fletcher, 2010). If fast dynamic stretching increases muscle strength due to the PAPE mechanism, then Fast-Wide, in which the intervention was performed at the fastest speed, should increase the muscle strength of the hamstrings. Previous studies reported that speed, amplitude, and end-range forces applied by dynamic stretching influence spinal and alpha-motorneuron excitability (Burke et al., 1978; Fetz et al., 1979; Vujnovich and Dawson, 1994; Guissard et al., 2001; Opplert and Babault, 2018). The effects are attributed to a significantly inhibitory contribution from Golgi tendon organs (Burke et al., 1978; Vujnovich and Dawson, 1994; Opplert and Babault, 2018). The Golgi tendon organs respond mainly to rapid and large-amplitude stretching and to the end-range forces applied, by decreasing the motoneuron excitability (Burke et al., 1978; Vujnovich and Dawson, 1994). Therefore, it was suggested that 30 seconds of dynamic stretching at a fast speed and normal amplitude is useful for an increase in the muscle strength of the hamstrings, but dynamic stretching at a wide amplitude could inhibit the increment in the muscle strength. In the present study, ROM increased in Slow-Wide, Fast-Normal, and Fast-Wide but was not changed in Slow-Normal. In addition, the muscle-tendon unit stiffness of the hamstrings did not change in any intervention. Change in ROM is attributed to changes in stretching tolerance and muscle-tendon unit stiffness (Behm et al., 2016; Opplert and Babault, 2018). In the present study, passive torque at the maximum knee extension angle was used to assess stretching tolerance (Kataura et al., 2017; Takeuchi and Nakamura, 2020a; Takeuchi et al., 2021a; 2021d). Therefore, it was indicated that 30 seconds of dynamic stretching at fast speed or wide amplitude increased ROM due to an increment in stretching tolerance, but did not change the muscle-tendon unit stiffness of the hamstrings in either method. The results of the present study did not suggest why ROM did not change in Slow-Normal although the passive torque increased. However, other factors (e.g., muscle temperature and muscle activities) may be involved these results, and further examination is necessary. Previous studies reported that dynamic stretching at 60 rpm and normal amplitude (within ROM) for 120 (Mizuno, 2017) and 210 seconds (Mizuno and Umemura, 2016) did not change muscle-tendon unit stiffness although dynamic stretching for 300 seconds significantly decreased it (Iwata et al., 2019). Moreover, dynamic stretching performed at the last 5 degrees of ROM for 120 seconds significantly decreases muscle-tendon unit stiffness (Konrad et al., 2017). These data indicated that the duration and amplitude of dynamic stretching are important factors to decrease muscle-tendon stiffness. In previous studies regarding static stretching, stretching duration and intensity are related to change in the muscle-tendon unit stiffness of the hamstrings, and longer (more than 180 seconds) (Matsuo et al., 2013; Nakamura et al., 2019) or higher intensity (120%ROM) (Kataura et al., 2017; Takeuchi and Nakamura, 2020a; 2020b; Takeuchi et al., 2021a; 2021b; 2021c) of stretching effectively decrease it. The minimum duration, speed, and amplitude of dynamic stretching required to decrease the muscle-tendon unit stiffness of the hamstrings are not known. However, it is possible that the 30 seconds of dynamic stretching used in sports settings may not be useful in a decrement in the muscle-tendon unit stiffness regardless of the speed and amplitude of the stretch. The present study examined the speed and amplitude of dynamic stretching. However, the load of dynamic stretching was not measured. In static stretching, it was found that a load of static stretching exerted on the hamstrings differs for each subject, even if the static stretching is performed under the same conditions (same duration and intensity), and the applied load is related to the decrease in the muscle-tendon unit stiffness of the hamstrings (McCrary et al., 2015). The applied load of dynamic stretching should be influenced by its speed, amplitude, and duration. It is necessary to quantify the applied load of dynamic stretching and to examine the relationship between the load and changes in flexibility and muscle strength after dynamic stretching.
Only 30 seconds of dynamic stretching at fast speed and normal amplitude increased the muscle strength of the hamstrings. No intervention changed the muscle-tendon unit stiffness of the hamstrings.
ACKNOWLEDGEMENTS |
This work was supported by JSPS KAKENHI with Grant number 22K11595.The experiments complied with the current laws of the country in which they were performed. The authors have no conflicts of interest to declare. The datasets generated and analyzed during the current study are not publicly available but are available from the corresponding author who was an organizer of the study. |
|
AUTHOR BIOGRAPHY |
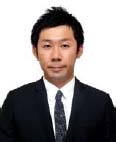 |
Kosuke Takeuchi |
Employment: Lecture, Department of Physical Therapy, Faculty of Rehabilitation, Kobe International University, Hyogo, Japan |
Degree: PhD |
Research interests: Sports science, stretching, sports medicine |
E-mail: ktakeuchi@kobe-kiu.ac.jp |
|
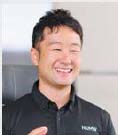 |
Masatoshi Nakamura |
Employment: Associate professor, Faculty of Rehabilitation Sciences, Nishi Kyushu University, Saga, Japan |
Degree: PhD |
Research interests: Physical therapy, stretching, exercise physiology, flexibility |
E-mail: nakamuramas@nisikyu-u.ac.jp |
|
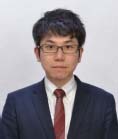 |
Shingo Matsuo |
Employment: Lecture, Department of Rehabilitation, Faculty of Health Sciences, Nihon Fukushi University, Aichi, Japan |
Degree: PhD |
Research interests: Stretching exercise and muscle flexibility |
E-mail: matsuo@n-fukushi.ac.jp |
|
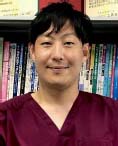 |
Kazunori Akizuki |
Employment: Lecture, Department of Physical Therapy, Mejiro University, Saitama, Japan |
Degree: PhD |
Research interests: Physical therapy, sports psychology, motor control, motor learning |
E-mail: k.akizuki@mejiro.ac.jp |
|
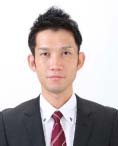 |
Takamasa Mizuno |
Employment: Associate Professor, Research Center of Health, Physical Fitness and Sports, Nagoya University, Aichi, Japan |
Degree: PhD |
Research interests: Stretching, Strength training, flexibility |
E-mail: mizuno@htc.nagoya-u.ac.jp |
|
|
|
REFERENCES |
 Bandy W.D., Irion J.M., Briggler M. (1997) The effect of time and frequency of static stretching on flexibility of the hamstring muscles. Physical Therapy 77, 1090-1096. Crossref
|
 Bandy W.D., Irion J.M., Briggler M. (1998) The effect of static stretch and dynamic range of motion training on the flexibility of the hamstring muscles. The Journal of Orthopaedic and Sports Physical Therapy 27, 295-300. Crossref
|
 Behm D.G., Blazevich A.J., Kay A.D., McHugh M. (2016) Acute effects of muscle stretching on physical performance, range of motion, and injury incidence in healthy active individuals: A systematic review. Applied Physiology, Nutrition and Metabolism 41, 1-11. Crossref
|
 Behm D.G., Chaouachi A. (2011) A review of the acute effects of static and dynamic stretching on performance. European Journal of Applied Physiology 111, 2633-2651. Crossref
|
 Behm D.G., Plewe S., Grage P., Rabbani A., Beigi H.T., Byrne J.M. (2011) Relative static stretch-induced impairments and dynamic stretch-induced enhancements are similar in young and middle-aged men. Applied Physiology, Nutrition, and Metabolism 36, 790-797. Crossref
|
 Burke D., Hagbarth K.E., Löfstedt L. (1978) Muscle spindle activity in man during shortening and lengthening contractions. The Journal of Physiology 277, 131-142.
|
 Cohen, J. (1988) Statistical Power Analysis for the Behavioral Sciences (2nd Ed.). Hillsdale, NJ Lawrence Erlbaum Associates, Publishers. - References - Scientific Research Publishing
|
 Fetz E.E., Jankowska E., Johannisson T., Lipski J. (1979) Autogenetic inhibition of motoneurones by impulses in group Ia muscle spindle afferents. The Journal of Physiology 293, 173-195. Crossref
|
 Fletcher I.M. (2010) The effect of different dynamic stretch velocities on jump performance. European Journal of Applied Physiology 109, 491-498. Crossref
|
 Fletcher I.M., Anness R. (2007) The acute effects of combined static and dynamic stretch protocols on fifty-meter sprint performance in track-and-field athletes. Journal of Strength and Conditioning Research 21, 784-787. Crossref
|
 Guissard N., Duchateau J., Hainaut K. (2001) Mechanisms of decreased motoneurone excitation during passive muscle stretching. Experimental Brain Research 137, 163-169. Crossref
|
 Hough P.A., Ross E.Z., Howatson G. (2009) Effects of Dynamic and Static Stretching on Vertical Jump Performance and Electromyographic Activity. Journal of Strength and Conditioning Research 23, 507-512. Crossref
|
 Iwata M., Yamamoto A., Matsuo S., Hatano G., Miyazaki M., Fukaya T., Fujiwara M., Asai Y, Suzuki S. (2019) Dynamic stretching has sustained effects on range of motion and passive stiffness of the hamstring muscles. Journal of Sports Science and Medicine 18, 13-20. Pubmed
|
 Jaggers J.R., Swank A.M., Frost K.L., Lee C.D. (2008) The acute effects of dynamic and ballistic stretching on vertical jump height, force, and power. Journal of Strength and Conditioning Research 22, 1844-1849. Crossref
|
 Judge L.W., Avedesian J.M., Bellar D.M., Hoover D.L., Craig B.W., Langley J. (2020) Pre- and Post-Activity Stretching Practices of Collegiate Soccer Coaches in the United State. International Journal of Exercise Science 13, 260.
|
 Kataura S., Suzuki S., Matsuo S., Hatano G., Iwata M., Yokoi K. (2017) Acute Effects of the Different Intensity of Static Stretching on Flexibility and Isometric Muscle Force. Journal of Strength and Conditioning Research 31, 3403-3410. Crossref
|
 Kaufman K.R., Brodine S.K., Shaffer R.A., Johnson C.W., Cullison T.R. (1999) The effect of foot structure and range of motion on musculoskeletal overuse injuries. The American Journal of Sports Medicine 27, 585-593. Crossref
|
 Konrad A., Stafilidis S., Tilp M. (2017) Effects of acute static, ballistic, and PNF stretching exercise on the muscle and tendon tissue properties. Scandinavian Journal Of Medicine & Science In Sports 27, 1070-1080. Crossref
|
 Magnusson S.P., Simonsen E.B., Aagaard P., Boesen J., Johannsen F., Kjaer M. (1997) Determinants of musculoskeletal flexibility: viscoelastic properties, cross-sectional area, EMG and stretch tolerance. Scandinavian Journal Of Medicine & Science In Sports 7, 195-202. Crossref
|
 Matsuo S., Iwata M., Miyazaki M., Fukaya T., Yamanaka E., Nagata K. (2019) Changes in Flexibility and Force are not Different after Static Versus Dynamic Stretching. Sports Med Int Open 23, 89-95. Crossref
|
 Matsuo S., Suzuki S., Iwata M., Banno Y., Asai Y., Tsuchida W. (2013) Acute Effects of Different Stretching Durations on Passive Torque, Mobility, and Isometric Muscle Force. Journal of Strength and Conditioning Research 27, 3367-3376. Crossref
|
 McCrary J.M., Ackermann B.J., Halaki M. (2015) A systematic review of the effects of upper body warm-up on performance and injury. British Journal Of Sports Medicine 49, 935-942. Crossref
|
 Mizuno T. (2017) Changes in joint range of motion and muscle-tendon unit stiffness after varying amounts of dynamic stretching. Journal of Sports Sciences 35, 2157-2163. Crossref
|
 Mizuno T. (2022) Effects of Dynamic Stretching Velocity on Joint Range of Motion, Muscle Strength, and Subjective Fatigue. Journal of strength and conditioning research 36, 2440-2447. Crossref
|
 Mizuno T., Umemura Y. (2016) Dynamic Stretching does not Change the Stiffness of the Muscle-Tendon Unit. International Journal Of Sports Medicine 37, 1044-1050. Crossref
|
 Morse C.I. (2011) Gender differences in the passive stiffness of the human gastrocnemius muscle during stretch. European Journal Of Applied Physiology 111, 2149-2154. Crossref
|
 Nakamura M., Ikezoe T., Nishishita S., Tanaka H., Umehara J., Ichihashi N. (2019) Static stretching duration needed to decrease passive stiffness of hamstring muscle-tendon unit. The Journal of Physical Fitness and Sports Medicine 8, 113-116. Crossref
|
 Opplert J., Babault N. (2018) Acute Effects of Dynamic Stretching on Muscle Flexibility and Performance: An Analysis of the Current Literature. Sports Medicine (Auckland, N.Z.) 48, 299-325. Crossref
|
 Pickering Rodriguez E.C., Watsford M.L., Bower R.G., Murphy A.J. (2017) The relationship between lower body stiffness and injury incidence in female netballers. Sports Biomechanics 16, 361-373. Crossref
|
 Sale D.G. (2002) Postactivation potentiation: role in human performance. Exercise and Sport Sciences Reviews 30, 138-143. Crossref
|
 Samukawa M., Hattori M., Sugama N., Takeda N. (2011) The effects of dynamic stretching on plantar flexor muscle-tendon tissue properties. Manual Therapy 16, 618-622. Crossref
|
 Takeuchi K., Akizuki K., Nakamura M. (2021a) Time course of changes in the range of motion and muscle-tendon unit stiffness of the hamstrings after two different intensities of static stretching. PLOS ONE 16, e0257367. Crossref
|
 Takeuchi K., Akizuki K., Nakamura M. (2021b) Association between static stretching load and changes in the flexibility of the hamstrings. Scientific Reports 11, 21778. Crossref
|
 Takeuchi K., Akizuki K., Nakamura M. (2021c) The acute effects of high-intensity jack-knife stretching on the flexibility of the hamstrings. Scientific Reports 11, 12115. Crossref
|
 Takeuchi K., Nakamura M. (2020a) Influence of High Intensity 20-Second Static Stretching on the Flexibility and Strength of Hamstrings. Journal of Sports Science & Medicine 19, 429-435. Pubmed
|
 Takeuchi K., Nakamura M. (2020b) The optimal duration of high-intensity static stretching in hamstrings. PLOS ONE 15, e0240181. Crossref
|
 Takeuchi K., Nakamura M., Kakihana H., Tsukuda F. (2019) A Survey of static and dynamic stretching protocol. International Journal of Sport and Health Science 17, 72-79. Crossref
|
 Takeuchi K., Sato S., Kiyono R., Yahata K., Murakami Y., Sanuki F. (2021d) High-Intensity Static Stretching in Quadriceps Is Affected More by Its Intensity Than Its Duration. Frontiers in Physiology 12. Crossref
|
 Takeuchi K., Takemura M., Nakamura M., Tsukuda F., Miyakawa S. (2021e) Effects of Active and Passive Warm-ups on Range of Motion, Strength, and Muscle Passive Properties in Ankle Plantarflexor Muscles. Journal of Strength and Conditioning Research 35, 141-146. Crossref
|
 Vujnovich A.L., Dawson N.J. (1994) The effect of therapeutic muscle stretch on neural processing. The Journal of Orthopaedic and Sports Physical Therapy 20, 145-153. Crossref
|
 Watsford M.L., Murphy A.J., McLachlan K.A., Bryant A.L., Cameron M.L., Crossley K.M. (2010) A prospective study of the relationship between lower body stiffness and hamstring injury in professional Australian rules footballers. The American Journal Of Sports Medicine 38, 2058-2064. Crossref
|
 Yamaguchi T., Ishii K. (2005) Effects of static stretching for 30 seconds and dynamic stretching on leg extension power. ournal of Strength and Conditioning Research 19, 677-683. Crossref
|
 Yamaguchi T., Ishii K., Yamanaka M., Yasuda K. (2007) Acute Effects of Dynamic Stretching Exercise on Power Output During Concentric Dynamic Constant External Resistance Leg Extension. The Journal of Strength and Conditioning Research 21, 1238-1244. Crossref
|
|
|
|
|
|
|