|
|
|
ABSTRACT |
Dynamic stretching (DS) is a conditioning technique commonly used in sports. However, its effects on contralateral joint flexibility and elastic properties of the targeted muscle over several weeks remain unclear. Similarly, its impact on muscle functions, such as force or power exertion, is poorly understood. This study aimed to examine the effects of a 12-week DS program targeting the ankle plantar flexors on the joint flexibility, muscle strength, and elastic properties of the plantar flexors in stretched and contralateral legs. Eighteen healthy young adults (12 males and six females, aged 18-24 years) participated in this study. DS was performed on one leg of the ankle plantar flexors for 12 weeks (3 days weekly), while the other leg served as a non-intervention. Dorsiflexion range of motion (DFROM), muscle stiffness, and ankle plantar flexion strength (rate of torque development and isokinetic muscle strength) were assessed before and after the intervention. The muscle stiffness was measured using shear wave elastography on the gastrocnemius. DFROM significantly increased in both conditions (p < 0.05), whereas gastrocnemius stiffness significantly decreased only in the DS condition (p < 0.05). No significant changes were observed in the non-intervention condition. The ankle plantar flexion rates of torque development and isokinetic strength did not change in either condition. These findings suggest that 12 weeks of DS can reduce muscle stiffness and improve joint flexibility without affecting muscle strength while enhancing contralateral joint flexibility without altering muscle stiffness or strength. |
Key words:
Muscle elasticity, stiffness, range of motion, muscle strength, shear wave elastography, training effect
|
Key
Points
- This study aimed to examine the effects of a 12-week dynamic stretching program targeting the ankle plantar flexors on the joint flexibility, muscle strength, and elastic properties of the plantar flexors in stretched and contralateral legs.
- This study demonstrated that a 12-week dynamic stretching program for ankle plantar flexors improved dorsiflexion range of motion in intervention and non-intervention legs.
- The findings of this study suggest that 12 weeks of dynamic stretching can reduce muscle stiffness and improve joint flexibility in the intervention leg without affecting muscle strength and enhance joint flexibility in the non-intervention leg without altering muscle stiffness or strength.
|
Dynamic stretching (DS), including ballistic stretching, involves moving joints through their maximum or submaximal range of motion (ROM) approximately once per second, with repeated dynamic muscle lengthening and shortening. It is commonly used for warming up in sports settings and has been shown to improve joint flexibility without reducing muscle functions, such as maximal muscle strength and explosive power exertion (Bacurau et al., 2009; Sekir et al., 2010; Yamaguchi and Ishii, 2014; Mizuno and Umemura, 2016). Moreover, ankle dorsiflexion ROM (DFROM) has been shown to improve after six weeks of continuous DS of the ankle plantar flexors (Mahieu et al., 2007; Konrad and Tilp, 2014). Although a review study indicated that DS may be less effective than static stretching for this purpose on the stretched side (Konrad et al., 2024), DS enhanced joint flexibility. Static stretching, in which muscles are stretched without recoil and held in position for a sustained duration (less than 60 s is recommended to avoid performance deficits), is a well-established method for improving flexibility (Behm et al., 2016). Notably, continuous static stretching for several weeks enhances the flexibility of stretched joints (Freitas et al., 2018; Medeiros and Martini. 2018; Konrad et al., 2024), as well as in the contralateral, non-performing limb by reporting a systematic review (Behm et al., 2021; Nakamura et al., 2022). The increased limb flexibility during stretching intervention is attributed to reduced muscle stiffness and changes in neurophysiological factors (Behm et al., 2016). In the non-intervention limb, flexibility improvements are thought to result from neural inhibition and psychophysiological factors, such as increased stretch tolerance, rather than changes in passive muscle-tendon properties, including muscle stiffness (Behm et al., 2016; Behm et al., 2021). As DS can improve flexibility on the intervention side, it is expected to have similar effects on the non-intervention side. However, the impact of prolonged DS on flexibility and muscle stiffness in the non-intervention limbs remains unclear. A previous systematic review reported that static stretching training produced small and significant improvements in muscle functions for intervention limbs (Thomas et al., 2023). Moreover, a previous study reported that 10 weeks of continuous static stretching can improve muscle function in intervention and non-intervention legs (Nelson et al., 2012). Thus, if muscle stretch training improves the muscle functions of the intervention side, it is expected that the muscle functions of the non-intervention side will also improve. However, there is no consensus on whether DS enhances muscle function on the intervention side (Turki-Belkhiria et al., 2014; Barbosa et al., 2020). If DS enhances muscle function on the intervention side, it could also impact the non-intervention side; nonetheless, this is yet to be confirmed. This study aimed to investigate the effects of a 12-week DS program targeting the ankle plantar flexors on joint flexibility, muscle stiffness, and muscle strength in intervention and non-intervention legs. We hypothesized that a 12-week DS program would decrease muscle stiffness and improve joint flexibility in the intervention limbs while enhancing contralateral joint flexibility without altering muscle stiffness.
ParticipantsEighteen healthy students (12 males and six females; males: age, 20 ± 2 years; height, 1.72 ± 0.07 m; body weight, 64.9 ± 11.1 kg; females: age, 20 ± 1 years; height, 1.61 ± 0.08 m; body weight, 56.1 ± 5.5 kg; mean ± standard deviation) participated in this study. All participants had no orthopedic diseases of the lower extremities (muscle, tendon, or ligament injuries or peripheral neuropathy). In addition, they engaged in regular exercise at least once per week. They were instructed not to begin any new training program targeting lower-extremity strength or flexibility during the study period. Informed consent was obtained from all participants after they were provided with details of the purpose, procedures, benefits, and risks of the study. This study was reviewed and approved by the Ethical Review Committee for Human Subjects Research of the authors’ institution (approval number: BKC-LSMH-2023-009).
Study designThis study evaluated the effects of a 12-week DS program targeting the ankle plantar flexors on joint flexibility, muscle stiffness, and muscle strength in intervention and non-intervention groups. Both legs were measured in all participants. One leg underwent DS intervention, while the other did not. The intervention leg was randomly assigned, regardless of dominance. Measurements were collected for both legs in the same order before and after the intervention. Dependent variables included DFROM, muscle stiffness, and ankle plantar flexion strength (ankle plantar flexion rate of torque development [RTD] and isokinetic strength).
Dynamic stretching protocolThe DS undertook stretching of the plantar flexors in a standing wall push position following the one described by a previous study (Konrad and Tilp, 2014). The participants performed two types of DS exercises for the ankle plantar flexor group in a standing position: (1) flexion and extension of the ankle joint in a narrow ROM at 1.5 Hz and (2) flexion and extension in a wide ROM at 1 Hz (https://jssm.org/video/IkedaVideo1a.html and https://jssm.org/video/IkedaVideo1b.html). For the latter, the participants were instructed to raise their heels to an angle of approximately 45° between the plantar surface and floor. Based on a previous study (Konrad and Tilp 2014), both DS exercises were performed with discomfort intensity (4 on a visual analog scale of 1-5, 1: no pain at all, 2: mild stretching feeling, 3: moderate stretching feeling, 4: discomfort, and 5: intolerable discomfort). Two types of DS exercises were performed three times weekly for 12 weeks, with each session consisting of five sets of 60 s per set for each DS type (interval between sets, 30 s).
Measurement of Dorsiflexion range of motionThe DFROM was assessed using an isokinetic dynamometer (BIODEX SYSTEM 4; Biodex Medical Systems, Shirley, NY, USA) (Figure 1). The participants assumed a posture with the knee joint fully extended, the hip flexed to 60°, and the ankle positioned at 30° plantar flexion (0°: anatomical position). The thighs and feet were secured using straps. The ankle was passively dorsiflexed at a speed of 2°/s until the participants felt moderate stretching (visual analog scale score of 3) (Ikeda et al., 2024). DFROM was defined as the angle from 0° to the maximum dorsiflexion angle during measurement. This measurement was performed five times, and the mean of the three measurements was used to represent the DFROM. During the measurements, the participants were instructed to remain relaxed and not resist passive dorsiflexion. The torque and ankle joint angle signals from the isokinetic dynamometer were converted at 1 kHz using an analog-to-digital (A/D) converter (PowerLab/16SP, ADInstruments, Sydney, Australia) and recorded on a personal computer using analysis software (LabChart, v8.1.2, ADInstruments).
Measurement of muscle stiffnessShear wave elastography (Aixplorer, MSK mode; SuperSonic Imagine, France) was used to measure the shear modulus of the medial gastrocnemius (MG) as an indicator of muscle stiffness. For MG measurements, the ankle joint angle was set to 0° and 10° of dorsiflexion based on a previous study (Ikeda et al., 2024). The participants were seated with their knees fully extended as in the DFROM measurements and secured to an isokinetic dynamometer. MG measurements were obtained at the center of the muscle belly (approximately 30% proximal to the length of the lower leg). A linear ultrasound probe (scan width: 50 mm; SL10-2, SuperSonic Imagine, France) was positioned at the measurement site, and long-axis B-mode ultrasound images with color-mapping shear modulus values were captured. Five images were acquired for each joint angle, and the participants were instructed to remain relaxed. Ultrasound images were analyzed using elastography image analysis software (T.K.K. 5840, Takei Scientific Instruments, Japan) (Figure 2). A rectangular region of interest (ROI) was placed on each image, and the mean shear modulus within the ROI was recorded. The MG stiffness for each joint angle was calculated as the mean of three shear modulus values, excluding the largest and smallest readings.
Measurement of ankle plantar flexion strengthThe RTD for isometric plantar flexion and isokinetic ankle plantar flexion strength was measured using a previously described dynamometer with the participants seated. For the RTD measurement, the participants kept their knees fully extended, and the ankle joint was fixed at 0° on the footplate of the dynamometer. Before the RTD measurement, the participants performed a warm-up that produced a force of 30%, 50%, and 80% of their maximal effort. The isometric plantar flexion RTD was measured five times at 60-s intervals. Participants were instructed to generate torque “as fast and powerfully as possible” (Maffiuletti et al., 2016; Ikeda et al., 2021). The torque and ankle joint angle signals from the isokinetic dynamometer were converted at 1 kHz using an A/D converter (PowerLab/16SP, ADInstruments, Sydney, Australia) and recorded on a PC using an analysis software (LabChart8, v8.1.2, ADInstruments). The torque signal was smoothed using a fourth-order zero-lag digital Butterworth filter with a 15 Hz cutoff frequency (Aagaard et al., 2002; Ikeda et al., 2021). The RTD is defined as the maximum time derivative of the torque waveform for each trial. The average RTD value was calculated from three trials, excluding the highest and lowest values. The isokinetic ankle plantar flexion strength was measured at angular velocities of 60°/s and 180°/s with maximal effort. The testing position was the same as that used for the RTD measurements. The ROM was set from 0° to 40° plantar flexion, and the joint was returned to the starting position after each movement. Three trials were performed at each angular velocity (interval between trials: 60 s), and the highest recorded value was used for the analysis.
Statistical analysisAll data were presented as mean ± standard deviation. Two-way repeated-measures analysis of variance (ANOVA; IBM SPSS Statistics 24, SPSS Japan, Tokyo, Japan) was performed on the pre-and post-intervention data in the intervention condition (DS and no intervention) × time (pre- and post-intervention). If an interaction or main effect of time was observed, paired t-tests were performed for each condition. A paired t-test was also performed to confirm that there were no significant differences in the pre-intervention measures between the conditions. Cohen’s d (post-hoc comparison) and partial η2 (ηp2: ANOVA) were used to calculate the effect size. Cohen’s d was obtained using the following equation: d = Mdiff/SDpooled √2[1 - r], where Mdiff is the difference between the mean value of the pre-and post-measurements, and r is the correlation between the mean values (Morris and DeShon, 2002). A pre-study statistical power analysis indicated a sample size of 14 participants per condition (within-factor repeated-measures analysis of variance, effect size = 0.4, power = 0.8, alpha level = 0.05) (Cohen, 1988) using G* Power 3. The effect size (Cohen’s d) was defined as: |0.20| - |0.50| small effect, |0.50| - |0.80| medium effect, and >|0.80| large effect (Cohen, 1988). Statistical significance was set at p < 0.05.
The intraclass correlation coefficient (ICC) values for each measurement variable were 0.80 or more, indicating good reliability: DFROM, 0.93; MG stiffness at 0°, 0.96; MG stiffness at dorsiflexion 10°, 0.97; RTD of isometric plantar flexion, 0.86; isokinetic ankle plantar flexion strength at an angular velocity of 60°/s, 0.83; isokinetic ankle plantar flexion strength at an angular velocity of 180°/s, 0.81. The coefficient of variation values for each measurement variable were as follows: DFROM, 1.1%; MG stiffness at 0°, 3.2%; MG stiffness at dorsiflexion 10°, 3.2%; RTD of isometric plantar flexion, 13.5%; isokinetic ankle plantar flexion strength at an angular velocity of 60°/s, 10.5%; and isokinetic ankle plantar flexion strength at an angular velocity of 180°/s, 12.5%. A significant interaction between condition and time was observed for DFROM (p = .029; ηp2 = 0.25), with post-hoc tests indicating significant large magnitude increases in the DS and moderate magnitude increases in the non-intervention conditions, respectively (DS: 8.7 ± 7.4° [Pre], 14.4 ± 9.0° [Post]; p < .001; d = 1.04; non-intervention: 8.7 ± 8.6° [Pre], 11.8 ± 10.2° [Post]; p = .022; d = 0.63) (Figure 3). No interaction between the condition and time or main effect of time was found for MG stiffness at an ankle joint angle of 0 ° (Table 1). However, a significant main effect of time was observed for MG stiffness at 10° ankle dorsiflexion (p = .016; ηp2 = 0.30). Post-hoc tests revealed a significant large magnitude decrease in MG stiffness in the DS condition (p = .006; d = 0.80); in contrast, no significant small magnitude change was observed in the non-intervention condition (p = 0.19; d = 0.33) (Table 1). For the RTD of isometric plantar flexion, no significant interaction between condition and time or primary effect of time was detected (Table 2). An interaction between condition and time was observed for isokinetic ankle plantar flexion strength at an angular velocity of 60°/s (p = .025; ηp2 = 0.26). However, post-hoc tests showed no significant small magnitude changes in DS or non-intervention conditions (DS: p = 0.24; d = 0.31; non-intervention: p = 0.19; d = 0.33) (Table 2). Additionally, no interaction between condition and time or primary effect of time was found for the isokinetic ankle plantar flexion strength at an angular velocity of 180°/s (Table 2).
This study demonstrated that a 12-week DS program for ankle plantar flexors improved DFROM in intervention and non-intervention legs. However, a reduction in gastrocnemius stiffness was observed only in the intervention legs. In addition, no significant changes were detected in the RTD for isometric plantar flexion or isokinetic ankle plantar flexion strength in either leg of the post-intervention measures. After the intervention, the observed improvement in DFROM in the DS group suggests that the DS protocol effectively enhanced joint flexibility. Previous studies have shown that DS can improve joint flexibility with intervention periods lasting 6 weeks or more (Mahieu et al., 2007; Konrad and Tilp, 2014). Another study reported that static stretching performed for 3 to 8 weeks did not alter muscle or tendon stiffness but increased extensibility and tolerance to tensile loading (Freitas et al., 2018). Therefore, the 12-week DS protocol was chosen to assess its potential to reduce muscle stiffness. Interestingly, DFROM also improved in the non-intervention legs. Furthermore, 4 weeks of continuous static stretching has been shown to improve joint flexibility on the contralateral side (Nakamura et al., 2022), and the results suggest that continuous DS may have a similar effect. Whether DS can improve contralateral joint flexibility within an intervention period of less than 12 weeks requires further study. Joint flexibility improvements from muscle stretching are often attributed to reduced stiffness in the muscle-tendon unit and changes in neurophysiological factors, such as stretch tolerance (Behm et al., 2016). In this study, gastrocnemii stiffness was reduced under DS conditions. This result suggests that decreased muscle stiffness owing to DS training contributes to improved flexibility. A previous study reported no reduction in gastrocnemius stiffness after six weeks of DS (Konrad and Tilp, 2014), indicating that shorter DS interventions may be insufficient to reduce stiffness. Further research is needed to clarify the minimum effective duration of DS on muscle stiffness. Although neurophysiological changes were not directly assessed in this study, they may have influenced the improvement in DFROM under DS conditions. In the non-intervention leg, no reduction in gastrocnemius stiffness was detected, implying that the improved DFROM in this leg was likely owing to neurophysiological adaptations rather than changes in muscle stiffness. Previous studies reported that static stretching training can increase contralateral joint flexibility without altering muscle stiffness (Behm et al., 2021; Nakamura et al., 2022). Thus, regardless of the stretching modality, muscle stretching training for several weeks is thought to increase joint flexibility in the contralateral side by changing factors other than muscle stiffness. Further studies are required to clarify whether these factors, such as the neurophysiological or mechanical properties of tendons, change by DS training. On the other hand, there was a tendency for the effect size of DFROM in DS condition (d = 1.04: large effect) to be larger than that in non-intervention condition (d = 0.63: medium effect). This could be explained by a significant decrease in muscle stiffness in DS condition only. Therefore, muscle stretching seems to be necessary to decrease muscle stiffness. Previous studies have reported mixed findings on the effect of DS training on muscle function, with some showing improvements and others showing no change (Turki-Belkhiria et al., 2014; Barbosa et al., 2020). This study found no significant changes in the RTD or isokinetic ankle plantar flexion strength under DS conditions, which supports previous findings of no improvement in muscle function. Similarly, no increase in muscle strength was observed in non-performing legs in the present study. A previous study reported that 10-week static stretching improved contralateral muscle strength (Nelson et al., 2012). In this previous study, the muscle strength in the intervention leg also increased, suggesting that static stretching was an intensity that provided a training stimulus sufficient to increase the force production ability of the participants. However, a systematic review reported that the non-local effects of prolonged static stretching reveal that both the stretched and contralateral, non-stretched limbs of young adult participants demonstrate, on average, small magnitude strength deficits (Behm et al., 2021). In other words, if there is not enough stimulation to increase strength on the stretched side, the non-stretched side will not increase strength either. Here, DS may not have provided sufficient stimuli to enhance the strength of the active young participants. Further research is needed to assess the effects of DS training in populations with low activity levels. This study did not include surface electromyography measurements, which made the muscle activity levels uncertain during the intervention. Additionally, it is unknown whether similar effects would be observed in different muscle groups, particularly the proximal groups that may transmit stimulation across the trunk. Future studies should investigate the effects of DS on more proximal muscle groups, such as knee flexors. Furthermore, because this study focused on young, healthy participants, the findings may not be generalizable to populations with different characteristics, such as children, older adults, and athletes. Finally, differences in the muscle stiffness assessment methods may have contributed to the discrepancies between the findings of the present study and those of Konrad and Tilp (2014), who reported no effect of continued DS use on stiffness. Future research should consider the impact of varying assessment methods when interpreting the results.
This study demonstrated that a 12-week DS program for ankle plantar flexors improved DFROM in intervention and non-intervention legs. However, a reduction in gastrocnemius stiffness was observed only in the intervention legs. Additionally, no significant changes were detected in the RTD for isometric plantar flexion or isokinetic ankle plantar flexion strength in either leg before or after the intervention.
ACKNOWLEDGEMENTS |
The experiments comply with the current laws of the country where they were performed. The authors have no conflict of interest to declare. The datasets generated during and/or analyzed during the current study are not publicly available but are available from the corresponding author who organized the study. This study was supported by JSPS KAKENHI (Grant-in-Aid for Young Scientists, grant number 22K17704). |
|
AUTHOR BIOGRAPHY |
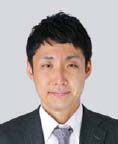 |
Naoki Ikeda |
Employment: Institute for General Education, Ritsumeikan University, Japan |
Degree: PhD |
Research interests: Biomechanics, muscle mechanics and exercise physiology |
E-mail: nikeda305@gmail.com |
|
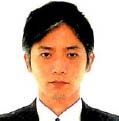 |
Kazuya Hiratsuka |
Employment: Faculty of Health Care, Hachinohe Gakuin University, Japan |
Degree: MSc |
Research interests: Biomechanics, muscle mechanics and exercise physiology |
E-mail: hrtk@hachinohe-u.ac.jp |
|
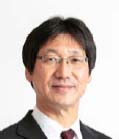 |
Tadao Isaka |
Employment: Faculty of Sport and Health Science, Ritsumeikan University, Japan |
Degree: PhD |
Research interests: Biomechanics, muscle mechanics and training |
E-mail: isaka@se.ritsumei.ac.jp |
|
|
|
REFERENCES |
 Aagaard P., Simonsen E.B., Andersen J.L., Magnusson P., Dyhre-Poulsen P. (2002) Increased rate of force development and neural drive of human skeletal muscle following resistance training. Journal of Applied Physiology 93, 1318-1326. Crossref
|
 Bacurau R.F., Monteiro G.A., Ugrinowitsch C., Tricoli V., Cabral L.F., Aoki M.S. (2009) Acute effect of a ballistic and a static stretching exercise bout on flexibility and maximal strength. Journal of Strength and Conditioning Research 23, 304-308. Crossref
|
 Barbosa G.M., Trajano G.S., Dantas G.A.F., Silva B.R., Vieira W.H.B. (2020) Chronic Effects of Static and Dynamic Stretching on Hamstrings Eccentric Strength and Functional Performance: A Randomized Controlled Trial. Journal of Strength and Conditioning Research 34, 2031-2039. Crossref
|
 Behm D.G., Alizadeh S., Anvar S.H., Drury B., Granacher U., Moran J. (2021) Non-local Acute Passive Stretching Effects on Range of Motion in Healthy Adults: A Systematic Review with Meta-analysis. Sports Medicine 51, 945-959. Crossref
|
 Behm D.G., Blazevich A.J., Kay A.D., McHugh M. (2016) Acute effects of muscle stretching on physical performance, range of motion, and injury incidence in healthy active individuals: a systematic review. Applied Physiology, Nutrition, and Metabolism 41, 1-11. Crossref
|
 Cohen, J. (1988) Statistical Power Analysis for the Behavioral Science.
2nd ed. Hillsdale: Lawrence Erlbaum Associates.
|
 Freitas S.R., Mendes B., Le Sant G., Andrade R.J., Nordez A., Milanovic Z. (2018) Can chronic stretching change the muscle-tendon mechanical properties? A review. Scandinavian Journal of Medicine & Science in Sports 28, 794-806. Crossref
|
 Ikeda N., Hiratsuka K., Isaka T. (2024) Effect of 6-Week Instrument-Assisted Soft Tissue Mobilization on Joint Flexibility and Musculotendinous Properties. Sports (Basel) 12, 150. Crossref
|
 Ikeda N., Yonezu T., Kawakami Y. (2021) Minute oscillation stretching: A novel modality for reducing musculo-tendinous stiffness and maintaining muscle strength. Scandinavian Journal of Medicine & Science in Sports 31, 104-114. Crossref
|
 Konrad A., Alizadeh S., Daneshjoo A., Anvar S.H., Graham A., Zahiri A., Goudini R., Edwards C., Scharf C., Behm D.G. (2024) Chronic effects of stretching on range of motion with consideration of potential moderating variables: A systematic review with meta-analysis. Journal of Sport and Health Science 13, 186-194. Crossref
|
 Konrad A., Tilp M. (2014) Effects of ballistic stretching training on the properties of human muscle and tendon structures. Journal of Applied Physiology 117, 29-35. Crossref
|
 Maffiuletti N.A., Aagaard P., Blazevich A.J., Folland J., Tillin N., Duchateau J. (2016) Rate of force development: physiological and methodological considerations. European Journal of Applied Physiology 116, 1091-1116. Crossref
|
 Mahieu N.N., McNair P., De Muynck M. M., Stevens V., Blanckaert I., Smits N., Witvrouw E. (2007) Effect of static and ballistic stretching on the muscle-tendon tissue properties. Medicine and Science in Sports and Exercise 39, 494-501. Crossref
|
 Medeiros D.M., Martini T.F. (2018) Chronic effect of different types of stretching on ankle dorsiflexion range of motion: Systematic review and meta-analysis. Foot (Edinburgh, Scotland) 34, 28-35. Crossref
|
 Mizuno T., Umemura Y. (2016) Dynamic Stretching does not Change the Stiffness of the Muscle-Tendon Unit. International Journal of Sports Medicine 37, 1044-1050. Crossref
|
 Morris S.B., DeShon R.P. (2002) Combining effect size estimates in meta-analysis with repeated measures and independent-groups designs. Psychological Methods 7, 105-125. Crossref
|
 Nakamura M., Yoshida R., Sato S., Yahata K., Murakami Y., Kasahara K., Fukaya T., Takeuchi K., Nunes J.P., Konrad A. (2022) Cross-education effect of 4-week high- or low-intensity static stretching intervention programs on passive properties of plantar flexors. Journal of Biomechanics 133, 110958. Crossref
|
 Nelson A.G., Kokkonen J., Winchester J.B., Kalani W., Peterson K., Kenly M.S., Arnall D.A. (2012) A 10-week stretching program increases strength in the contralateral muscle. Journal of Strength and Conditioning Research 26, 832-836. Crossref
|
 Sekir U., Arabaci R., Akova B., Kadagan S.M. (2010) Acute effects of static and dynamic stretching on leg flexor and extensor isokinetic strength in elite women athletes. Scandinavian Journal of Medicine & Science in Sports 20, 268-281. Crossref
|
 Thomas E., Ficarra S., Nunes J.P., Paoli A., Bellafiore M., Palma A., Bianco A. (2023) Does stretching training influence muscular strength? A systematic review with meta-analysis and meta-regression. Journal of Strength and Conditioning Research 37, 1145-1156. Crossref
|
 Turki-Belkhiria L., Chaouachi A., Turki O., Chtourou H., Chtara M., Chamari K., Amri M., Behm D.G. (2014) Eight weeks of dynamic stretching during warm-ups improves jump power but not repeated or single sprint performance. European Journal of Sport Science 14, 19-27. Crossref
|
 Yamaguchi T., Ishii K. (2014) An optimal protocol for dynamic stretching to improve explosive performance. Journal of Physical Fitness and Sports Medicine 3, 121-129. Crossref
|
|
|
|
|
|
|