|
|
|
ABSTRACT |
This study aimed to (1) determine the kinematics of the different body parts during the execution of one of the basic combinations of plays – a few selected strokes and the footwork used to combine them, and (2) evaluate the variation of these kinematics. The study included eight male advanced-level (polish national team) table tennis players (aged 22.7 ± 2.7 years). All of them were right-handed and presented an offensive style of play. Participants performed one task: performing a series of topspin strokes. Kinematic parameters were measured using the MR3 myoMuscle Master Edition system. The movement of the playing hand was used to assess specific cycle events. Angular values and velocities of the body segments and maximal linear velocities of the playing hand were calculated. The basic statistics were applied. Quartile coefficients of variation were used to assess the interindividual variability. The research made it possible to determine the kinematics of the strokes used in the combination of plays in table tennis. The essential elements of coordination of movements during the combination of three topspin strokes were indicated. Players make strokes during a series of plays at high frequency and velocity (up to several hundred degrees/s at some joints), reducing the ranges of motion in specific body segments, which were observed to be smaller (even up to several dozen degrees) than the analogous ranges reported in the literature for single strokes. This seems to be a significant note to players and coaches in table tennis. In addition, the players execute the stroke slightly differently than in single strokes, which is caused by the need to combine the striking movement with footwork and quickly position themselves for subsequent hits. The players also used different, variable execution patterns (different angles – settings in the joints), although the effect – in the form of the maximum velocity of the playing hand – was very similar. This can be considered a manifestation of a phenomenon called “functional movement variability,” which means that athletes adjust their movements using different movement patterns but achieve similar results regarding maximum linear hand speed. This highlights the importance of the individualisation process in table tennis. |
Key words:
Sports, movement coordination, movement variability, complex sport technique
|
Key
Points
- The research carried out in this work allowed to determine the kinematics of combination strokes - a combination of three different topspin strokes
- The most important elements of coordination of movements during the tasks performed, that is, the combination of three different topspin strokes, are indicated.
- The study found that players make strokes during a series of plays at high velocity, reducing the ranges of motion in specific body segments.
- In the strokes performed in the series, the players used different execution patterns (different angles – settings in the joints), although the effect – in the form of the maximum velocity of the playing hand – was very similar. This can be considered a manifestation of a phenomenon called “functional movement variability,” which means that athletes adjust their movements, using different movement patterns, but achieve similar results in terms of maximum linear hand speed.
|
Table tennis is a very diverse game when it comes to the technical and tactical actions taken by the players, and their type largely depends on the player’s abilities and skills, as well as the opponent, the game situation, gender, etc. (Grycan and Bańkosz, 2024). The game of table tennis is one of the fastest sports (Biz et al., 2022), in which movement technique (strokes and their combinations, footwork, etc.) is essential for reaching the championship level (Wu, 2017). Therefore, describing and obtaining data on the technique is of inestimable importance. However, the range of issues related to table tennis technique seems enormous. This is because players use many techniques in different variations, depending on the strength, speed, and direction of movement used, in different playing situations, placing the ball differently with a certain velocity and rotation on the table, with the possibility of inter- and intra-individual variability in the playing technique used. The information coming from evaluating the biomechanics of strokes and footwork in table tennis seems very important. The study of table tennis kinematics has become increasingly popular and widespread in recent years, undoubtedly aided by the development of technology and testing tools. Among the most frequently addressed problems in this field are those concerning the determination of the kinematics of the body’s individual parts during the performance of strokes in table tennis (Inno and Kojima, 2009; 2011; Malagoli Lanzoni et al., 2018). Some studies concerned table tennis footwork (Qian et al. 2016, He et al. 2021) and the kinematics of the racket during the most frequently used strokes (Bańkosz and Winiarski, 2017). Research in this field usually concerns the comparison of kinematics between athletes of different levels of sport (Wang et al., 2018, He et al., 2021). Sometimes, researchers address the problem of the relationship between the movements of selected body segments and the racket (Iino et al., 2008; Bańkosz and Winiarski, 2018). In the literature, studies that compare the execution of strokes with different racket grips can be found (Xia et al., 2020). Most of the studies indicated above, however, do not consider the differences between different variations of the technique of the same stroke, which, as described above, can have different values of the force used, the rotation given to the ball, or the velocity (e.g., fast topspin “from the table” vs. strong topspin from half distance). The issue of movement variability has intrigued researchers and sports practitioners for years. It is an essential element occurring during free movements, which some regard as movement “noise” not worthy of scientific consideration and others as a normal phenomenon, manifesting functional variability, injury avoidance, and adaptation (Bartlett et al. 2007). Often, the variation in movement is due to a compensation mechanism – a change in the range of motion in one joint, for example, is compensated for by a change in another (Dupuy et al., 2000). The authors emphasise that even the best athletes experience variability when performing the same movement (Bartlett et al., 2007). Movement variability occurs in sports activities due to the extreme complexity of the neuromusculoskeletal system and the redundancy of its degrees of freedom (Bartlett et al., 2007; Preatoni et al., 2012; Hamill et al., 2006). Cowin et al. (2022) distinguish three types of movement variability: strategic variability (describes the approaches or methods of movement used to complete a task), execution variability (describes the intentional and unintentional adjustments of the body between repetitions within the same strategy); and outcome variability (describes the differences in the result or product of a movement). Assessing inter-individual or intra-individual variability can be important for sports theorists and practitioners. Thanks to its understanding, it is possible, for example, to create alternative movement programs with a recommendation to perform a given movement or elements of sports technique. Its consideration seems essential in individualising training, tailoring the training program to the athlete’s needs (Dupuy et al., 2000). The evaluation of movement variability in table tennis is poorly researched. Bootsma and van Wieringen (1990) evaluated variation in racket motion during forehand drive stroke, confirming functional variation in racket kinematic parameters. They also pointed out less variation in the spatial dimension (direction of the racket’s movement) when it contacted the ball. Similar observations were made by Sheppard and Li (2007), who studied the parameters of racket movement during a forehand drive stroke, finding a decreasing variation in some of them when the racket contacted the ball, emphasising the importance of controlling the movement during its execution. A recent study by Iino et al. (2017) also found that the ability to exploit the racket angle’s alignment (stabilisation) limitation at the moment of ball contact may be a key element in determining the game’s effectiveness. Recent studies (Bańkosz and Winiarski, 2020; Bańkosz et al., 2020) indicate large intra- and inter-individual variations in kinematic parameters during the execution of a topspin forehand stroke. As indicated above, the study of kinematics and its variability is most often concerned with a single technique (of a given stroke, comparison of several strokes, determination of kinematics of a single step, etc.). In real-game situations, however, the so-called complex technique is much more common, i.e., combining two or more strokes into a combination of strokes. And it is the compound technique - the combinations of strokes and the footwork that enables them to be combined - reflects the game situation. No studies evaluate the kinematics of the strokes used in the compound technique or the degree of variation. Evaluating the kinematics of the compound technique presented by high-level athletes in table tennis and demonstrating its variability can allow, as mentioned above, to show some models of the performance of strokes and their combinations and the range of their variability. This information can be helpful to coaches and table tennis players and allow them to bring it into training for competitions. In addition, the finding of a significant variation in the kinematics of the used strikes may emphasise the need to apply the principle of individualisation in the training process, especially regarding sports technique and its teaching (e.g., creating alternative movement programs). Therefore, this study aimed to determine the kinematics of the different body parts during the execution of one of the basic combinations of plays – a few selected strokes and the footwork used to combine them and evaluate the variation of these kinematics.
ParticipantsThe study included eight advanced-level male table tennis players (from the Polish national team), aged 22.7 ± 2.7 years, with a height of 178 ± 2.7 and a body weight of 76 ± 7.4 kg. All participants were right-handed, offensive players with over 10 years of competitive experience. The participants were informed of the purpose of the study and gave informed consent to participate in the experiment. The Bioethics Committee approved the experiment (The Senate Bioethics Commission of Wroclaw University of Health and Sport Sciences, approval no. 34/2019).
ProceduresThe tests were performed at the Table Tennis Sports Centre in Grodzisk Mazowiecki. Before the tasks, every participant followed standardised warm-up procedures: general (15 minutes) and table tennis-specific (20 minutes). Participants performed one task, which consisted of performing a series of strokes sequentially: topspin backhand from the backhand corner (B), topspin forehand from the backhand corner after pivot (Fbh), and topspin forehand from the forehand corner (Ffh). This was task No. 52 (Falkenberg) from the set of exercises included in the Robo-Pong 2055 robot manual. This sequence (B, Fbh, and Ffh) was repeated five times; together, 15 strokes were presented. Players were asked to hit the marked area in both corners of the table (30x30 cm) diagonally (Instruction given: “Play diagonally, accurately, and as hard as you can.” Every successful shot considered “on the table” and played diagonally was recorded for further analysis (missed balls, balls hit out of bounds, balls hit into the net, etc.). were excluded). The balls were shot by a dedicated table tennis robot (Newgy Robo-Pong 2055 robot, Nevgy Industries, Tennessee, USA - Figure 1) at constant parameters of rotation, speed, direction, and flight trajectory (chosen task from the list, set automatically - task number 52, Figure 1). For the experiment, the same racket with the following characteristics was used: blade - Jonyer-H-AN (Butterfly, Japan), rubbers (both sides) - Tenergy 05, 2.1 mm (Butterfly, Japan). Plastic Andro Speedball 3S 40+ balls (Andro, Germany) and a Stiga Premium Compact table (Stiga, Sweden) were used.
Instruments and measurementsKinematic parameters were measured using the MR3 myoMuscle Master Edition system (myoMOTION™, Noraxon, USA – Figure 1). Noraxon’s Inertial Measurement Units can be considered an alternative to the optical motion capture system for movement analysis. The IMU 3D angular measurement showed mostly good to high test-retest reliability with relatively small standard error of measurement (Tao et al., 2012). During dynamic trials, the MSE (root mean squared error) for MyoMotion, when compared with Vicon, is expected to be 0.50, and the correlation coefficient between Vicon and MyoMotion for dynamic trials to be 0.99 (Balasubramanian, 2013). The accuracy and validity of the IMU system in angle determination is unquestioned and was a subject of previous research (Sharif Bidabadi et al., 2018). The myoMOTION system consists of a set of (1 to 16) sensors using Inertial Sensor technology. Based on so-called fusion algorithms, the information from a 3D accelerometer, gyroscope, and magnetometer is used to measure the 3D rotation angles of each sensor in absolute space (Yaw-pitch-roll, also called orientation or navigation angles). Inertial sensors were located on the body of the study participant to record the accelerations, according to the myoMotion protocol described in the manual (Figure 1). Sensors were attached by the same technician with unique straps and elastic self-adhesive tape. Every strap had its pocket for the inertial sensor. The straps with the sensors were light and easy to use and wear. The sensors were placed symmetrically so that the positive x-coordinate on the sensor label corresponded to a superior orientation for the trunk, head, and pelvis (Figure 1). Every participant, at the beginning of the measure, was checked, and the system was calibrated. The positive x-coordinate corresponded to a proximal orientation for the limb segment sensors. For the foot sensor, the x-coordinate was directed distally (to the toes). The sensors were placed according to the myoMotion manual protocol. The max sampling rate for a given sensor/receiver was 100 Hz per sensor for the whole 16-sensor set. The following angles were recorded: ankle dorsiflexion, ankle inversion, ankle abduction, knee flexion, hips flexion, hips abduction, hips rotation, lumbar rotation, lumbar flexion, lateral lumbar bending, thoracic rotation, thoracic flexion, thoracic lateral bending, playing-hand shoulder: flexion, abduction and rotation, playing-hand elbow flexion, playing-hand wrist: extension, supination, and radial abduction. The movement of the playing hand was used to assess specific cycle events. The designated events were 0 (start of B), 1 (end of B), 2 (start of Fbh), 3 (end of Fbh), 4 (start of Ffh) and 5 (end of Ffh). Measurements of the non-playing limb were omitted. When analysing the kinematics of the phases, only the evaluation of the forward phases, i.e., the hitting phases, which were the time segments 0-1 (hitting phase B), 2-3 (hitting phase Fbh), and 4-5 (hitting phase Ffh), were included in the study. This paper calculated angular values in events, angular velocities in events, maximum angular velocities in forward (hitting) phases, and maximum linear velocities of the playing hand in forward (hitting) phases.
Statistical analysisStatistical calculations were performed using Statistica 13.1 (TIBCO Software Inc). The Shapiro-Wilk test was used to test the normality of data distribution for each variable (angular parameters, velocities). A lack of normality in data distribution was found. The basic statistics were applied. The assessment of interindividual variability of individual parameters consisted of calculating quartile coefficients of variation and evaluating them. An interpretation was adopted as for the classical CV, recognising that the higher the value of the coefficient, the greater the variation, also using the interpretation: < 25% - low variability; 25%-45% - average variability; 45%-100% - substantial variability; > 100% - extreme variability.
Angle values in each event and coordination of movementsThe results of the angular values and the descriptive statistics about them can be seen in Figure 2, Figure 3, Figure 4, Figure 5, and Table 1. Angular velocities and descriptive statistics are presented in Table 2. By analysing the individual figures and comparing them with each other, it is possible to trace the range of motion and its direction in each joint in the entire group of subjects. During the execution of topspin backhand (B), the players perform a flexion movement at the ankle joints in the hitting phase (on average about 20 degrees, angular velocities approx. 100 - left (L) - 160 - right (R) deg/s), right knee joint extension (average approx. 15 degrees, max velocity L - 180 deg/s, R - 320 deg/s), minimal extension in the left knee, slight extension of the trunk and its lateral flexion (to the right) - Figure 3, Figure 4, Table 1, Table 2. The shoulder joint of the playing limb in the hitting phase shows flexion (range on average about 50 degrees, maximum angular velocity 388 deg/s), abduction (range average 40 degrees, velocity 399 deg/s), and external rotation (average 50 degrees, max. velocity - 638 deg/s) - Figure 2, Table 1, Table 2. The extension was observed at the elbow joint during the hitting phase (approx. 40 degrees, max. velocity - 142 deg/s). At the wrist joint in the discussed phase, the movements of extension, radial flexion, and supination of the hand are noticed, with an average range of motion of approx. 15, 20, and 50 degrees, respectively. The maximum angular velocities in these movements are 534, 286, and 1046 deg/s, respectively). The resultant maximum linear velocity of the playing hand in the hitting phase is 7.5 m/s. (Table 3). The combination of B with the second stroke, a topspin forehand after a pivot from the backhand (bh) corner (Fbh), required a change in the alignment of the entire body. The athletes put their right leg backwards, flexing it at the right hip joint and rotating it outward. These movements range from 20-30 degrees (Figure 3, Figure 4, Table 1). The alignment in the joints of the right foot is like that in B. A trunk rotation to the right follows (range approx. 20 degrees), and flexion to the right (approx. 15 degrees) is necessary. The hitting phase of Fbh involves trunk extension, i.e., hip extension and lumbar extension (on average several degrees) and rotation to the left (approx. 10 degrees, Figure 5, Table 1) and about 20 degrees of extension in the right knee joint, slight flexion in the left. This movement corresponds to the centre of gravity transfer from the right leg to the left. The maximum angular velocities are, in this phase, approx. 65 deg/s (hip), 22 deg/s (lumbar), about 11-14 deg/s, and in the knee joint – 180 deg/s (L) - 360 deg/s (R) degrees/s (Table 2). The upper limb in the hitting phase is flexed at the shoulder joint to an average of approx. 50 degrees (maximum velocity of 100 deg/s), deferred in a range of several degrees (max velocity of 88 deg/s and rotated inward in a range of approx. 30 degrees, with a maximum angular velocity of this movement of 40 deg/s) - Figure 2, Table 1, Table 2. At the elbow joint of the playing limb, the flexion of approx. Thirty degrees on average, with a maximum angular velocity of 94 deg/s, is observed. The wrist joint is flexed radially in the striking phase of Fbh in a range of several degrees (maximum velocities of 23 and 11 deg/s, respectively), and the hand in several degrees of supination motion. The maximum resultant linear velocity of the playing hand in this phase is 5.44 m/s (Table 3). Higher values were noticed for the upward and forward components (9 m/s). The last stroke of the cycle is a topspin forehand from the forehand (fh) corner (Ffh). In the joints of the ankle, knees, and hips at the onset of the stroke, one notices a similar alignment to that of Fbh, with the difference seen in less external rotation in the hips in the case of Ffh (Figure 3, Figure 4, Table 1). The player rotates the hips to approx. 15 degrees for alignment. For Ffh players, position the torso similarly to Fbh, with slightly less external rotation. The playing limb is placed along the torso, in slight (20 degrees) adduction and external rotation, with approx. 30-degree flexion at the elbow joint and an almost intermediate position at the wrist joint, with slight extension and elbow flexion, 5-10 degrees (Figure 2, Table 1). In the hitting phase of Ffh, slight flexion is observed in the ankle joints, slight flexion in the left knee joint and extension in the right, an extension in the right hip joint, and considerable flexion and rotation in the left hip joint (60 degrees and 30 degrees, respectively), with angular velocities of maximum: ankles - left (L) -14 deg/s, right (R) - 40 deg/s; knee joints - in both ca. 70 deg/s; hip flexion - L - 65 deg/s, R - 56 deg/s. Slight extension, left flexion, and left rotation can be observed in the trunk (Figure 5). The magnitudes of the maximum angular velocity reach several deg/s in these movements (Table 2). The movements of the playing limb in the hitting phase of the Ffh are flexion at the right shoulder joint (range approx. 80 degrees, angular velocity max - approx. 97 deg/s), abduction (20-30 degrees, max velocity - 61 deg/s), and internal rotation at this joint (average 40 degrees, velocity - 33); flexion at the right elbow joint (30-40 degrees, max velocity - approx. 70 deg/s). In the wrist joint in the described phase, approx. Ten degrees of flexion and hand supination, with maximum angular velocities of 14 and 32, respectively, were observed (Figure 2, Table 1, Table 2). The average maximum resultant linear velocity of the playing hand in this stroke (median) is 5.5 m/s (Table 3). Higher values were recorded for the upward and forward components. The Ffh stroke is combined with the B stroke. In the transition to this stroke, the players take a step to the left, positioning themselves similarly to the Ffh position as far as the angles of the lower limb joints are concerned, making a slight movement in the direction of placing the torso at 0 degrees and reducing its rotation to the left. Players prepare the playing limb for the next stroke by extension, adduction, and rotation at the shoulder joint. At the elbow joint, the playing limb remains in flexion (approx. 60 degrees); it is flexed at the wrist joint in the range of 40 degrees, towards the elbow (movement opposite to radial flexion) in the range of approx. 20-30 degrees, and the hand is supinated to approx. 40 degrees. In the setting described above, the player is prepared for stroke B.
Assessment of inter-individual variabilityThe values of quartile deviation and quartile coefficient of variation (qCV) can be seen in Table 1, Table 2, and Table 3. The tremendous values of qCV (from tens to hundreds of %) in torso positioning in individual events are noteworthy. However, the values of quarter deviations are a few to several degrees (Table 1). The most significant values are seen in lateral flexion and trunk flexion, especially at the end of B and the beginning and end of Fbh. Small values are noted for both parameters in the alignment (angles) of the hip joints in each event, but this does not apply to the alignment in rotation in both hip joints. The position of abduction in the right hip joint and flexion in the left in the Ffh onset event is also highly variable, although it should be remembered that the value of the angles is low in these cases. High qCV values (often reaching several hundred per cent) and several degrees of quarter deviation were noted in the settings at the ankle joints in each event (Table 1). Knee joint alignment in individual events is characterised by several degrees of variation and often a variation of less than 40% (qCV values). This does not apply to the last event in the cycle, in which the coefficient of variation in the alignment of the knee joints reaches 50-60%, and the variation is more than 30 degrees (Table 1). The right shoulder joint alignment is characterised by relatively low variation estimated from qCV (except for rotation at the end of B - 145%, the end of Fbh and the beginning and the end of Ffh, and extension at the beginning of Fbh and Ffh) and deviations of a dozen or more degrees (Table 1). Alignment in the right elbow joint corresponds to a small variation in individual events; the quarter deviation is usually 20-30 degrees, and qCV is a few tens of per cent, from 20 to 90%. The alignment of the right wrist joint in radial flexion movement is characterised by wide variation, as evidenced by values of quarter deviation often above 20 degrees and qCV values of several hundred per cent (Table 1). Extension of the right wrist joint in individual events is characterised by slightly less variation than radial flexion. However, qCV values vary in the 70-140% range, except in the last event, where more than 300% was recorded (Table 1). Right-hand movements are values of several degrees of deviation and several hundred per cent qCV in most events (Table 1). The study also evaluated the variation in the values of maximum angular velocities in the hitting (forward) phase. During this phase, high values of angular velocity variation, from 60 to 130%, can be observed in the trunk movements during the B stroke, even higher in the ankle joints. In the knee and hip joints, as well as the right shoulder and elbow, the variation is smaller, reaching several tens of per cent (except for abduction in the shoulder - 120%) - Table 2. The Fbh and Ffh hitting phases are characterised by less variation in maximum angular velocities than B, there are more values up to tens of per cent. The values of linear velocities of the playing hand show little and average variation among the study group (Table 3).
The purpose of this study was to evaluate kinematic values of topspin stroke sequences (a so-called complex technique, which reflects real game situations more than individual shots) - angles and angular velocities at the joints in specific events, the maximum angular velocity at these joints, the maximum linear velocity of the playing hand in the hitting phase, and to assess the level of variability in the measured kinematic values. The aim of the study was also to assess the differentiation of these kinematics.
Observation and analysis of the stroke techniqueDuring the tests performed, the strokes presented by the players were performed at a high pace, with a small time interval between each ball, according to the rhythm imposed by the robot. As a result, the execution of strokes took place in time-scarce conditions, thus forcing players to play fast, “at the table.” In addition, the players demonstrated compound technique, a combination of three different strokes using adequate footwork. This was supposed to bring the experiment closer to real-game situations than the evaluation of individual shots. Analysing the changes in angles in successive events in the various body segments made it possible to evaluate the technique of executing individual strokes. The evaluation of sports technique in this study confirms some data indicated in the literature for single strokes (topspin forehand, topspin backhand (Bańkosz and Winiarski, 2017; 2018; Iino et al., 2008; Iino and Kojima 2009; 2011; He et al., 2021, Qian et al., 2016). What draws attention is the coordination of striking movements, in which multiple body segments are used – from the ankle joints (feet) to the hand of the playing limb in each stroke. Important movements in the coordinated kinematic chains demonstrated in the present study include stroke-specific movements. During B in the hitting phase, it includes extension at the knee and hip joints, abduction and external rotation at the shoulder joint, extension at the elbow joint, radial flexion and extension at the wrist joint, and supination of the hand. During the hitting phase of Fbh, the specific movements are right knee joint extension, hip joint abduction (mainly right, which positions the player more laterally relative to the table), left trunk rotation, shoulder joint flexion, and elbow joint flexion. The hitting phase of Ffh-specific movements is flexion at the left knee and left hip joint and increased abduction at the right hip joint (which illustrates the shift of the centre of gravity to the left leg and facilitates the execution of the movement with the right leg to initiate the movement with a step back and forth to the left), rotation of the trunk to the left, flexion, abduction and internal rotation at the right shoulder joint and flexion at the elbow joint. What seems peculiar is that the ranges of stroke movements performed in many cases are smaller than the analogous ranges reported in the literature for single strokes, presumably performed with great force. For example, in a previous study, Bańkosz and Winiarski (2018) report that the range of motion of trunk rotation in the hitting phase of topspin forehand is more than 130 degrees, flexion-extension at the knee joints are ranges of more than 40 degrees, flexion at the shoulder joint - more than 100 degrees, flexion at the elbow joint - approx. 70 degrees. In the present study, in analogous strokes, these values are trunk rotation in a range of several tens of degrees, in the knee joints - 10-20 degrees, in the shoulder joint - only at Ffh - approx. 70 degrees at the elbow joint - approx. 40 degrees. It is likely that in the present study, the players, given less time and faced with the need to complete the task quickly, reduced their range of motion, thereby probably generating slightly less force but maintaining the pace of play. This observation is an important tip for coaches and players on how to play fast and close to the table. This information can help coaches and players work on optimal movement, velocity, and power adjustments during fast play close to the table. Reducing the range of movement and the power generated are the most important elements of fast play in situations when there is not enough time and it is necessary to combine strokes.
Observation and analysis of the footwork techniqueAlso specific to the conditions of the tests performed (high velocity of combining strokes) are some movements from the range of footwork that facilitate the linking of strokes; for example, at the end of B (event 1), a flexion in the left knee joint, enabling a faster transition (presumably the execution of a pivot) to Fbh, followed by a greater flexion at the hip joints and a rotation of the trunk to the right at Fbh (event 2), facilitating the execution of this stroke. The large and pronounced shift of body weight to the left leg at the end of the Ffh (event 5) also allows a faster transition to the left step. This alignment in the joints of the lower limbs, which is primarily conducive to fast movement and not necessarily to generate maximum force (which requires a stable position), is an important element that should be recognised by coaches and players when perfecting the fast game. In addition, the presented method of footwork may indicate a certain table tennis technique model in which the player adapts movement to the conditions of fast play.
Analysis of body segments’ velocitiesThe study evaluated and determined the values of maximum angular velocity in the hitting phase in the studied joints and movements. Of note are the very high values of this velocity in the arm’s external rotation motion during a B-stroke. This may be a confirmation of the observations available in the literature, emphasising the importance of this movement for the velocity of the racket in this stroke (Iino et al., 2008; Bańkosz and Winiarski, 2021; Bańkosz et al., 2020). Large values in this joint also apply to the flexion movement of the arm. Maximum angular velocities also reach high values in movements at the wrist joint: radial flexion, palm extension, and supination (in this movement, the angular velocity value is the highest) or rotation at the hip joints. These are probably the most important components of stroke movement during topspin bh. The maximum linear velocity of the hand (resultant) in this stroke reaches a value of nearly 8 m/s. This appears to be of low value compared to literature data on topspin bh (Bańkosz and Winiarski, 2017; Iino et al., 2008; Xia et al., 2020). The reason may be, as mentioned earlier, the timing conditions of the task performed by the players in this study, typical of a fast-paced, close-to-the-table game. In the Fbh hitting phase, the highest angular velocity values were found for movements at the shoulder joint, but the values were not as high as for B. Perhaps this is related to the inverted position and the need to travel some distance to assume the playing position. Similarly, the small value of the maximum linear velocity of the playing hand in the striking motion lower than at B and lower than indicated in the literature can be interpreted (Zhang and Shi, 2000). The duration of the hitting phase specified in the paper is also shorter than that reported by researchers in the literature (Zhang et al., 2016). The values for ranges of motion and angular velocities in the Ffh stroke are also slightly lower than for the two previously discussed strokes. The linear velocity of the racket and the duration of the forward phase in this stroke have a similar value to the Fbh stroke. This confirms previous observations and some literature data about adapting techniques to fast game conditions and using shorter movements (Malagoli Lanzoni et al., 2021) while generating less force. Used close to the table, the fast strokes thus have the character of strokes that “borrow” energy from the opponent (Chinese: “jièlì”). This is another important piece of information, like the ones mentioned above, that can help coaches and players as they work on adjusting the power and other parameters of their strokes in the game.
Analysis of the movement variabilityThe quartile index of variation was used as a measure of movement variability (Bonett, 2006; Botta-Dukát, 2023; Kornfeind et al., 2015; Reed et al., 2002). Analysing the variation in the alignment of individual body segments at the joints in each event, the very high coefficient of variation values for the trunk and ankle joints are noteworthy. The lower values apply to the knee, hip, right shoulder (out of rotation position), and elbow joints. Arguably, settings with less variation in the group indicate elements in the technique that are more constant and repeatable. It can also be noted that in situations of high dynamics, such as reaching Ffh, the variation in hand positioning is greater than in other events, indicating greater individual differences between players. Understanding this can help to provide more player-specific table tennis training. Despite the large variation in the alignment of body segments at different joints in individual events, characteristic features are smaller variations in the maximum values of angular velocities and small variations in maximum linear velocities in the hitting phase in individual strokes. As indicated in the literature, the moment of occurrence of the maximum linear velocity of the hand is the moment of close contact between the racket and the ball (Bańkosz and Winiarski, 2017; Fritsch et al., 2022). This moment appears crucial in a given stroke’s entire kinematic chain. The results obtained in the present study indicate that the large variation in alignment at the joints corresponds to a smaller variation in the maximum angular velocities achieved and a small variation in the maximum linear velocities of the playing hand. Thus, despite the large variation in the alignment of body segments at different joints in individual events, the characteristic features are smaller variations in the maximum values of angular velocities and small values of variation in the maximum linear velocities in the hitting phase in individual strokes (at the most important moment, which is the moment of stroke). Thus, one may be tempted to conclude that in the strokes performed in the series, the players used different execution patterns (different angles – settings in the joints), although the effect – in the form of the maximum velocity of the last member of the kinematic chain – was very similar. This may be a confirmation of previous observations (Bańkosz and Winiarski, 2020) and can be considered a manifestation of the phenomenon called functional movement variability (Cowin et al., 2022; Stergiou and Decker, 2011; Preatoni et al., 2012). It should be remembered that this differentiation may also result from the different body heights of the players, different limb lengths, body proportions, etc. It boils down to the fact that the task requirements in this research (dynamics, velocity, scarcity of time), like match situations, cause players to adjust their movements and use different movement patterns but achieve similar results. These highlight the importance of the individualisation process in table tennis. This individualisation may involve training the player in natural and typical game situations, selecting different opponents and sparring partners, considering the player’s current capabilities, etc. By remembering the principle of individualisation, trainers can conduct an optimal and accurate training process.
LimitationsA limitation of the work that needs to be acknowledged may be that the players studied, although they are the national elite of table tennis players, are not among the world’s top players. Therefore, the observations made would have to be confirmed by conducting similar tests on maximum sporting-level players. A similar limitation may result from the fact that the players come from only one country, so they do not represent a cross-section of many training systems. The research also involved a task performed under relatively constant and repeatable conditions. In addition, the work did not evaluate the real game but an exercise reflecting its conditions - a series of specific hits. Perhaps tests under different, variable, and unexpected playing conditions would yield different conclusions. One should also consider the limitations of the coefficient of variation used to assess kinematic variation, as indicated in the literature (Reed et al., 2003).
The research carried out in the study made it possible to determine the kinematics of the combination of strokes in table tennis, which reflect real game situations more than individual shots. The most important elements of coordination of movements during the tasks performed, that is, the combination of three different topspin strokes, were indicated. The study found that players make strokes during a series of plays at high velocity, reducing the ranges of motion in specific body segments. This makes it likely that they are not playing at maximum strength. In addition, the characteristics of the footwork indicate that the players are looking to execute the stroke as quickly as possible and move on to the next move, coordinating striking movements with footwork in a slightly different way than in single strokes. This seems to be a very important note to the coaches and players. In the strokes performed in the series, the players used different, variable execution patterns (different angles - settings in the joints), although the effect - in the form of the maximum velocity of the playing hand - was very similar. This can be considered a manifestation of a phenomenon called functional movement variability, which means that athletes adjust their movements using different movement patterns but achieve similar results in terms of maximum linear hand speed. This highlights the importance of the individualisation process in table tennis.
ACKNOWLEDGEMENTS |
The experiments comply with the current laws of the country where they were performed. The authors have no conflict of interest to declare. The datasets generated during and/or analyzed during the current study are not publicly available but are available from the corresponding author who organized the study. The project was co-financed by the Minister of Science and Higher Education in Poland under the Regional Excellence Initiative Programme |
|
AUTHOR BIOGRAPHY |
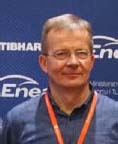 |
Ziemowit Bańkosz |
Employment: Department of Sports Didactics, Wrocław University of Health and Sport Sciences, Wrocław, Poland |
Degree: PhD |
Research interests: Table tennis sciences, kinematics, movement variability |
E-mail: ziemowit.bankosz@awf.wroc.pl |
|
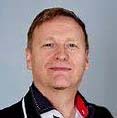 |
Sławomir Winiarski |
Employment: Department of Physiology and Biomechanics, Wrocław University of Health and Sport Sciences, Wrocław, Poland |
Degree: PhD |
Research interests: Biomechanics, movement analysis |
E-mail: Slawomir.winiarski@awf.wroc.pl |
|
|
|
REFERENCES |
 Bańkosz Z., Winiarski S. (2017) The kinematics of table tennis racquet: differences between topspin strokes. Journal of Sports Medicine and Physical Fitness 57, 202-213. Crossref
|
 Bańkosz Z., Winiarski S. (2018) Correlations between Angular Velocities in Selected Joints and Velocity of Table Tennis Racket during Topspin Forehand and Backhand. Journal of Sports Science and Medicine 17, 330-338. Pubmed
|
 Bańkosz Z., Winiarski S. (2020) Kinematic parameters of topspin forehand in table tennis and their inter- and intra-individual variability. Journal of Sports Science and Medicine 19, 138-148. Pubmed
|
 Bańkosz Z., Winiarski S. (2021) The application of statistical parametric mapping to evaluate differences in topspin backhand between Chinese and Polish female table tennis players. Applied Bionics and Biomechanics 14, 5555874. Crossref
|
 Bańkosz Z., Winiarski S., Malagoli Lanzoni I. (2020) Gender differences in kinematic parameters of topspin forehand and backhand in table tennis. International Journal of Environmental Research and Public Health 17, 5742. Crossref
|
 Balasubramanian, S. (2013) Comparison of angle measurements between
Vicon and Myomotion Systems. Master's thesis Center for
Adaptive Neural Systems (ANS), Arizona State University;
2013 Available from URL: https://pdfs.semanticscholar.org/1641/d6ece74c8e7e9e5f06595bad7b49540bc5
3a.pdf
|
 Bartlett R., Wheat J., Robins M. (2007) Is movement variability important for sports biomechanists?. Sports Biomechanics 6, 224-243. Crossref
|
 Biz C., Puce L., Slimani M., Salamh P., Dhahbi W., Bragazzi N.L., Ruggieri P. (2022) Epidemiology and risk factors of table-tennis-related injuries: Findings from a scoping review of the literature. Medicina (Kaunas) 58, 572. Crossref
|
 Bonett D.G. (2006) Confidence interval for a coefficient of quartile variation. Computational Statistics & Data Analysis 50, 2953-2957. Crossref
|
 Bootsma R.J., van Wieringen P.C.W. (1990) Timing an Attacking Forehand Drive in Table Tennis. Journal of Experimental Psy-chology: Human Perception and Performance 16, 21-29.
|
 Botta-Dukát Z. (2023) Quartile coefficient of variation is more robust than CV for traits calculated as a ratio. Scientific Reports 13, 4671. Crossref
|
 Cowin J., Nimphius S., Fell J., Culhane P., Schmidt M. (2022) A proposed framework to describe movement variability within sporting tasks: A scoping review. Sports Medicine Open 8, 85. Crossref
|
 Dupuy M., Mottet D., Ripoll H. (2000) The regulation of releaseparameters in underarm precision throwing. Journal of Sports Sciences 18, 375-382.
|
 Fritsch, C., Marsan, T., Poulet, Y., Thoreux, P., Rouch, P. and Sauret, C.
(2022) Is ball-racket contact concomitant with the maximal
racket speed in young trained table tennis players? In: Proceedings of 40th International Society of Biomechanics in
Sports Conference, Liverpool, UK: July 19-23, 2022. Ed: Robinson, M.A. Available from URL: https://commons.nmu.edu/cgi/viewcontent.cgi?article=2456&context=isbs
|
 Grycan, J. and Bańkosz, Z. (2024) The technical and tactical actions are
different styles of play in table tennis. Acta Kinesiologica
18(2), 41-55. Retrieved from https://akinesiologica.com/ojs_3.3.0-7/index.php/akinesiologica/article/view/224
|
 Hamill, J., Haddad, J.M., Heiderscheit, B.C., Van Emmerik, R.E.A. and
Li, L. (2006) Movement system variability. In: Movement System Variability Eds: Davids, K, Bennet S and Newell, K.
Champaign (IL): Human Kinetics. 153-166.
|
 He Y., Lyu X., Sun D., Baker J.S., Gu Y. (2021) The kinematic analysis of the lower limb during topspin forehand loop between different level table tennis athletes. PeerJ. 9, e10841. Crossref
|
 Iino Y., Kojima T. (2009) Kinematics of table tennis topspin forehands: Effects of performance level and ball spin. Journal of Sports Sciences 27, 1311-1321.
|
 Iino Y., Kojima T. (2011) Kinetics of the upper limb during table tennis topspin forehands in advanced and intermediate players. Sports Biomechanics 10, 361-377.
|
 Iino Y., Mori T., Kojima T. (2008) Contributions of upper limb rotations to racket velocity in table tennis backhands against topspin and backspin. Journal of Sports Sciences 26, 287-293.
|
 Iino Y., Yoshioka S., Fukashiro S. (2017) Uncontrolled manifold analysis of joint angle variability during table tennis forehand. Human Movement Science 56, 98-108.
|
 Kornfeind P., Baca A., Boindl T., Kettlgruber A., Gollnhu-ber G. (2015) Movement variability of professional pool billiards players on selected tasks. Procedia Engineering 112, 540-545.
|
 Malagoli Lanzoni I., Bartolomei S., Di Michele R., Fantozzi S. (2018) A kinematic comparison between long-line and cross-court top spin forehand in competitive table tennis players. Journal of Sports Sciences 36, 2637-2643. Crossref
|
 Malagoli Lanzoni I., Bartolomei S., Di Michele R., Gu Y., Baker J.S., Fantozzi S., Cortesi M. (2021) Kinematic analysis of the racket position during the table tennis top spin forehand stroke. Applied Sciences 11, 5178. Crossref
|
 Preatoni E., Hamill J., Harrison A. J., Hayes K., Van Emmerik R. E. A., Stokes C. W., Rodano R. (2012) Movement variability and skills monitoring in sports. Sports Biomechanics 12, 69-92.
|
 Qian J., Zhang Y., Baker J., Gu Y. (2016) Effects of performance level on lower limb kinematics during table tennis forehand loop. Acta of Bioengineering and Biomechanics 18, 149-155.
|
 Reed G. F., Lynn F., Meade B. D. (2002) Use of coefficient of variation in assessing variability of quantitative assays. Clinical and Vaccine Immunology 9, 1235-1239.
|
 Reed G. F., Lynn F., Meade B. D. (2003) Use of coefficient of variation in assessing variability of quantitative assays. Clinical and Vaccine Immunollogy 10, 1162. Crossref
|
 Sharif Bidabadi S., Murray I., Lee G.Y.F. (2018) Validation of foot pitch angle estimation using inertial measurement unit against marker-based optical 3D motion capture system. Biomedical Engineering Letters 8, 283-290.
|
 Sheppard A., Li F.X. (2007) Expertise and the control of interception in table tennis. European Journal of Sport Science 7, 213-222. Crossref
|
 Stergiou N., Decker L.M. (2011) Human movement variability, nonlinear dynamics, and pathology: is there a connection?. Human Movement Science 30, 869-888.
|
 Tao W., Liu T., Zheng R., Feng H. (2012) Gait analysis using wearable sensors. Sensors 12, 2255-2283.
|
 Wang M., Fu L., Gu Y., Mei Q., Fu F., Fernandez J. (2018) Comparative study of kinematics and muscle activity between elite and amateur table tennis players during topspin loop against backspin movements. Journal of Human Kinetics 15, 25-33. Crossref
|
 Wu, T.C. (2017) Core skills for world-level table tennis competitions. In:
The 14th ITTF Sports Science Congress and 5th World Racquet Sports Congress, April 23-25, Suzhou-China. Ed: Kondric, M., Xiaopeng, Z., Dandan, X. Book of Abstracts. 150-156
|
 Xia R., Dai B., Fu W., Gu N., Wu Y. (2020) Kinematic comparisons of the shakehand and penhold grips in table tennis forehand and backhand strokes when returning topspin and backspin balls. Journal of Sports Sciences and Medicine 19, 637-644. Pubmed
|
 Zagatto A.M., Gobatto C.A. (2012) Relationship between anaerobic parameters provided from MAOD and critical power model in specific table tennis test. International Journal of Sports Medicine 33, 613-620. Crossref
|
 Zagatto A.M., Papoti M., Gobatto C.A. (2008) Anaerobic capacity may not be determined by critical power model in elite table tennis players. Journal of Sports Sciences and Medicine 7, 54-59. Pubmed
|
 Zhang Z., Halkon B., Chou S. M., Qu X. (2016) A novel phase-aligned analysis on motion patterns of table tennis strokes. International Journal of Performance Analysis in Sport 16, 305-316. Crossref
|
 Zhang, H. and Shi, Y. (2000) Kinematics of forehand attack on accelerated loop techniques in table tennis - a case study. In: Proceedings of XVIII International Symposium on Biomechanics in
Sports, 2000 June 25-30; Hong Kong. Eds: Hong Y, Saunders
R, Johns DP. Department of Sports Science and Physical Education, The Chinese University of Hong Kong. 1011.
|
|
|
|
|
|
|