|
|
|
ABSTRACT |
Weekly training loads are typically reported using absolute values and are not individualized according to competition positional demands (relative values). The aim of this study was to evaluate absolute and relative training loads and compare across playing positions during a full in-season in an elite soccer academy. 24 elite academy soccer players, categorized into five positions (CD: central defender [n = 4]; FB: full back [n = 5]; CM: central midfielder [n = 6]; WM: wide midfielder [n = 5]; FW: forward [n = 4]), were monitored using a global positioning system. Absolute training load was calculated using the total distance, the distance at moderate-speed ([15-20[ km·h-1), high-speed ([20-25[ km·h-1), sprint (> 25 km·h-1), the total number of accelerations (> 3 m·s-2) and decelerations (< -3 m·s-2). Relative training load was calculated by dividing absolute training loads by mean values from the competitive matches. Training loads were determined daily according to their distance from match day (MD). One-way ANOVAs were performed to evaluate differences between playing positions. Absolute moderate-speed distance was greater for WM compared to CD (p = 0.015, and p = 0.017), while the opposite was shown for relative values (p = 0.014, and p < 0.001) on MD-4 and MD-3, respectively. The absolute moderate-speed distance was not different between CD, FB, CM, and FW, whereas relative values were greater for CD on MD+2 and MD-4 (p < 0.05). FB and WM performed greater absolute high-speed distance than CD on MD-4 and MD-3 (p < 0.05) while no difference was highlighted for relative values.Our results demonstrated that in the present academy players, training load for CD was underestimated using absolute training loads for moderate and high-speed distances. In contrast, relative training loads highlighted WM as an underloaded position. Therefore, relative training loads are recommended as they contextualize training loads according to competitive demands and favor training individualization. |
Key words:
GPS, microcycle, periodization, youth, high-intensity
|
Key
Points
- Relative loads should be quantified instead of absolute training loads to contextualize training loads based on players' demands during soccer competition.
- Relative training loads could help practitioners to further individualize training contents and improve prescription.
- Players should cover greater distances in high-velocity thresholds during training to replicate competitive demands.
- Practitioners should consider adding large-sided games and dissociated work to meet high-intensity requirements.
|
Global positioning systems (GPS) are commonly used across the majority of top-level team sports and particularly soccer (Akenhead and Nassis, 2016). Measuring external loads (e.g., distance, speed, accelerations) has widely helped our understanding of the work performed and capacities of athletes during training sessions and competition. For example, studies examining soccer competitions generally report that central midfielders cover the greatest total distance, wide midfielders and forwards cover the greatest high-intensity distance, while the central defender position is often reported as the least demanding (Akenhead et al., 2013; Bradley et al., 2009; Di Salvo et al., 2007; Mohr et al., 2003). These distinct positional demands during competitions have also been observed during training (Akenhead et al., 2016; Malone et al., 2015). However, quantitative analyses are generally conducted using absolute values (e.g., total distance covered at different velocities or the total number of accelerations). Although useful for training load monitoring, absolute values lack individualization. Indeed, for a similar external training load, players’ adaptative responses will differ based on their own physical capacities (Impellizzeri et al., 2019). Training load individualization can be achieved by adjusting for personalized physical capacities or using values for competitive physical demands. The latter has recently been proposed to calculate relative training loads by expressing absolute training loads as a fraction of players' competitive demands (Zurutuza et al., 2017). This alternative method has been used to describe the weekly microcycle (Baptista et al., 2019; Clemente et al., 2019; Guerrero-Calderón et al., 2022; Modric et al., 2021; Zurutuza et al., 2017). Studies have shown that the high-intensity competitive efforts were less replicated during training in comparison to low-intensity actions. However, to date, only two studies have explored possible positional differences (Baptista et al., 2019; Guerrero-Calderón et al., 2022). The authors highlighted that wide players (full backs and wide midfielders) were less likely to replicate their competitive demands, particularly during high-intensity efforts than wide back players (Baptista et al., 2019). In addition, while most positions underperformed in relation to their competitive high-intensity efforts, accelerations were shown to be overperformed (Guerrero-Calderón et al., 2022). Consequently, the authors proposed to modify training programmes to better match actual competition demands depending on positional demands (Guerrero-Calderón et al., 2022). Relative training loads would enable practitioners to individualize training loads and ensure that players are trained according to their competitive positional demands. Although the quantification of training loads using relative values is emerging, to the best of our knowledge, no study has attempted to compare the relative and absolute quantification methods to explore differences between playing positions during the training microcycle in academy soccer players. The aim of this study was to evaluate absolute and relative training loads and compare values across playing positions over the full in-season in an elite soccer academy. We hypothesized that these two methods of training load quantification would generate differences between positions by using absolute training loads (e.g., greater workload for wide as compared to central players) and that these positional differences would disappear when relative training loads are employed (i.e., training sessions similarly replicate the competitive demands for all positions).
ParticipantsTwenty-four elite academy soccer players (age = 18.2 ± 0.6 years, height = 177.8 ± 1.3 cm, body mass = 70.2 ± 3.9 kg) of the U19 team of the elite French Ligue 2 football club of Dijon Football Côte d’Or were recruited for this study. Physical capacities of the players (i.e., sprint, vertical jump and aerobic performances) are presented in Table 1. Players were categorized into five different positions: central defender (CD) (n = 4), fullback (FB) (n = 5), central midfielder (CM) (n = 6), wide midfielder (WM) (n = 5), and forward (FW) (n = 4). Goalkeepers were excluded from this study due to the different nature of their physical activity. All the players were notified of the research protocol, benefits, and risks before providing written informed consent in accordance with the declaration of Helsinki. Approval of the study was obtained from the local ethic committee (approval number: IRB00012476-2021-17-06-114).
DesignAn observational cohort study was conducted on an academy soccer team throughout the 2020/2021 season of the highest national youth soccer competition in France. The methodology was chosen in accordance with that previously used in similar studies (Clemente et al., 2019; Modric et al., 2021). Only the weeks that fulfilled the following criteria were considered: (i) the team played only one match during the week, (ii) a minimum of 6 days between matches, (iii) players completed all the training sessions in the week considered, and (iv) players should complete the entire match. Furthermore, to reduce the potential effects of situational and environmental factors, matches including red cards or specific contexts (e.g., bad weather, bad pitch, matches against teams that were mathematically assured of the title or relegation) were not analyzed (Chmura et al., 2021). As such, out of the 26 competitive weeks, 20 were included. This led to the average inclusion of 6.15 ± 0.97 players per week. Firstly, training loads (total distance, distances covered at specific speeds, and the number of accelerations and decelerations) were initially calculated as absolute values using GPS-derived data. Mean values were calculated daily from the considered weeks (see below). Secondly, relative training loads were calculated according to the demands identified in competitive matches. The mean values for all players’ competitive matches of the considered weeks were calculated for the same GPS-derived data (total distance, distances covered at specific speeds, and the frequency of accelerations and decelerations). On average, 4.9 ± 2.7 complete matches per player were used to calculate the mean values (CD: 6.4 ± 2.9 matches; FB: 5.7 ± 3.3; CM: 4.4 ± 2.8; WM: 4.25 ± 1.1; FW: 3.6 ± 1.0). Afterwards, absolute training loads were expressed as a function of the mean values of all the competitive matches of the considered weeks. Days were named according to their distance in days from matchday (MD) (Owen et al., 2017). For example, MD-4 corresponded to a training session four days before MD. The team followed a typical periodization throughout the season consisting of six technical-tactical field-based sessions and two gym-based sessions (Table 2). Only field-based sessions were analyzed (MD+2b, MD-4a, MD-4b, MD-3a, MD-2, MD-1) and gym-based sessions (MD+2a, MD-3b) were therefore excluded.
WorkloadPlayers wore a 10-Hz Fieldwizz GPS alongside a 100-Hz triaxial accelerometer microsensor on every field-based training session and match. This tracking system has shown low bias and good coefficient of variation for the total distance and peak speed, indicating high reliability to track team-sport variables (Willmott et al., 2019). The device was located between the scapulae using a special vest. As recommended by the manufacturer, all devices were activated 15 min before data collection. Players systematically wore the same sensor to avoid interunit variability. GPS were turned off as soon as the training session or match was stopped. Data were downloaded and analyzed immediately after each match and training session using the manufacturer’s propriety software package (Fieldwizz, ASI, Lausanne, Switzerland). The external load during each match and training session was monitored using the total distance, distance covered at specific speeds, frequency of accelerations, and decelerations. Total distance was the distance covered by the players and expressed in meters (m). Similarly, distances at specific speeds were expressed in m and included moderate-speed distance ([15-20[ km·h-1), high-speed distance ([20-25] km·h-1), and sprint distance (> 25 km·h-1). The total frequency of accelerations (> 3 m·s-2) and decelerations (< -3 m·s-2) was determined. Distances covered at each specific speed and the number of accelerations and decelerations, were determined as soon as the players reached the above thresholds and as long as the players remained within the given threshold. The absolute values, quantified during training, were subsequently expressed as a function of values obtained during competitive matches to compute relative training loads. In accordance with a previous study, the distance travelled at low-speed ([0-15[ km·h-1) was excluded from analyses due to its limited importance related to soccer performance (Clemente et al., 2019).
Physical testsPlayers performed physical tests at three different time points during the season. The tests included sprinting performance, vertical jump height and a maximal aerobic speed test. Tests were implemented at the end of the preseason (T1), just after the winter break (T2), and at the end of the season (T3). Two 30 m sprints were performed separated by 3 min of passive recovery. The 30 m sprinting times were measured using two pairs of photoelectric timing gates (Witty system, Microgate, Bolzano, Italy). Players started from a standing position as close as possible to the first timing gate. The best 30 m sprinting time was selected for analysis. Vertical jump height was evaluated using two counter movement jumps interspersed by 2 min of passive recovery. Players had to jump as high as possible from a standing position, then flexing the knees until 90° and extended the knees to jump in a continuous movement. They were asked to keep their arms on their hips from the standing position until landing. Performance was measured using a photocell jump system (Optojump, Microgate, Bolzano, Italy) sampling at 1000 Hz, with jump height (cm) subsequently calculated by proprietary software (Optojump, Version 1.3.20.0, Microgate, Bolzano, Italy). The best performance was retained for analysis. Maximal aerobic speed (MAS) was obtained using a 45 s/15 s incremental intermittent test on a track (45/15 MAS). The starting velocity was 8.0 km·h-1 and increased every 1 min with 0.5 km·h-1 increments. The last speed validated by the players was recorded as their MAS. All tests were performed by the same investigators, scheduled at the same time of day, performed in the same order, and using the same devices at each period (T1, T2, and T3). The mean value of the three different time points was used for analysis.
Statistical analysesAll statistical analyses were performed using JASP (Ver 0.13, JASP Team (2020), University of Amsterdam). Sphericity was examined by conducting the Mauchly’s test. One-way repeated measures analyses of variances (ANOVA) were performed to evaluate differences between positions (independent factor). These ANOVA were performed on physical tests. For each ANOVA, partial eta-squared (ηp2) was calculated as measures of effect size. Effect sizes were considered as small (<0.06), moderate (0.06-0.15), or large (>0.15) (18). In the case of significant position effects, Bonferroni’s post hoc tests were used to identify any localized effect. Mean values are presented with the effect size (Cohen’s d) and associated 95% confidence interval. Effect size was defined as trivial (<0.2), small (0.2-0.5), moderate (0.5-0.8) and large (>0.8) (18). The statistical significance was set at p < 0.05.
738 individual training sessions (CD = 192, FB = 204, CM = 132, WM = 102, FW = 108) and 123 individual matches (CD = 32, FB = 34, CM = 22, WM = 17, FW = 18) were analyzed.
Physical testsResults for physical tests are shown in Table 1 The ANOVAs revealed significant position effects for 30m (p < 0.001; ; ηp2= 0.609, large), CMJ (p = 0.014; ; ηp2= 0.449, large), and the 45/15 MAS (p = 0.004; ; ηp2 = 0.526). 30m time was lower for FB than CM (d = 2.702; p < 0.001), and FW (d = 2.279; p = 0.035). WM also demonstrated lower time than CM (d = 1.755; p = 0.012). CMJ demonstrated greater values for FB than CM (d = 2.046; p = 0.010). 45/15 MAS, was greater for CM and WM than FW (d = 2.437; p = 0.004, and d = 3.058; p = 0.029, respectively).
Competitive demandsIn match-play (Table 3), the ANOVAs revealed significant position effects for total distance (p < 0.001; ηp2 = 0.420, large), moderate-speed distance (p < 0.001; ηp2= 0.327, large), high-speed distance (p < 0.001; ηp2 = 0.497, large), sprint distance (p < 0.001; ηp2= 0.262, large), the frequency of accelerations (p < 0.001; ηp2 = 0.205, large) and decelerations (p < 0.001; ηp2= 0.339, large). Total distance was significantly greater for CM and WM than the other positions. Moderate-speed distance was significantly greater for WM compared to CD, FB, and CM. FW reported greater moderate-speed distance than CD and FB while CM covered greater moderate-speed distances than CD. High-speed distance was greater for WM versus the other positions. Both FB and FW demonstrated greater values than CD and CM. Sprint distance was greater for FB compared to CD, and CM. WM also covered greater sprint distance than CM. The frequency of accelerations and decelerations was greater for FB and WM than CD, and CM while FW also performed more decelerations than CD.
Training workloadSignificant main playing positional effects were obtained for all absolute and relative training load variables (Table S1). The absolute total distance was greater for WM compared to CM on MD+2b, CD and FB on MD-4b, and CD, CM and FW on MD-3a (Figure 1). FW also reported greater absolute total distance than FB on MD-4b. On MD-1, CM covered a greater absolute total distance than CD and FB. The relative total distance was greater for CD than CM on MD+2b, and MD-4a. On MD-4b, the relative total distance was greater for FW and WM than FB. The absolute moderate-speed distance was greater for WM than CM on MD+2b, CD, and FB on MD-4b, and CD on MD-3a (Figure 2). On MD-1, CM covered greater absolute moderate-speed distance than CD, and FB. The relative moderate-speed distance was greater for CD than the other positions on MD+2b, and MD-4a, WM on MD-4b, WM on MD-2, and FW on MD-1. A greater relative moderate-speed distance was also observed in FB than CM, and WM on MD+2b, WM, and FW on MD-4a, CM, WM, and FW on MD-3a, WM on MD-2, and WM on MD-1. CM covered a greater relative moderate-speed distance than WM on MD-1. The absolute high-speed distance was greater for WM than CD on MD+2b, MD-4a, and MD-3a (Figure 3). WM covered a greater absolute high-speed distance than CD on MD-4a, and MD-3a. The relative high-speed distance was greater in CM than the other positions on MD-4a. The absolute sprint distance was greater for FB than CD, and CM on MD+2b, and MD-2 (Figure 4). During MD-4a, and MD-3a, WM covered a greater absolute sprint distance than CD, and CM. The relative sprint distance was greater for FW than CD on MD-3a, and MD-2. The absolute number of accelerations was greater for FW, and WM than CM on MD-4a, and FB on MD-4b (Figure 5). FB, and WM, had greater absolute accelerations than CD, and CM on MD-3a. The absolute accelerations were greater for FB than CM on MD-2. FW performed a greater relative number of accelerations versus FB on MD-4b and MD-4a, and versus FB, and WM on MD-1. The relative frequency of accelerations was greater for CD compared to FB on MD-4a, and WM on MD-2. The absolute frequency of decelerations was greater for FB as compared to the other positions on MD-4a, and MD-3a, and CM, and FW on MD-2 (Figure 6). WM performed a greater frequency of absolute decelerations compared to the other positions on MD-4a, and MD-3a, CD, CM, and FB on MD-4b, and CD, CM, and FW on MD-1. The relative number of decelerations was greater for CD than FW on MD-4a, FB on MD-4b, and CM, WM, and FW on MD-2. On MD-4b, WM reported a greater relative number of decelerations than FB.
The aim of this study was to compare absolute versus relative external loads in training and compare these across playing positions over the full in-season in an elite soccer academy. The results only partly confirmed our a priori hypothesis. While WM performed greater workloads than CD and CM using the absolute training loads method, the analysis of relative training loads demonstrated that WM were underloaded compared to the other positions and that CD performed the greatest relative training loads. As such, these results suggest that quantifying relative training loads enables a more precise interpretation of players’ training loads compared to the absolute method. The main results regarding absolute training loads suggested that WM performed greater workloads on several days compared to the other positions for all GPS-derived values. Conversely, CD had the lowest training loads. Previous studies also obtained similar results in professional players (Akenhead et al., 2016; Malone et al., 2015; Owen et al., 2017). In addition, wide players (FB and WM) demonstrated a greater number of accelerations and decelerations during training compared to central positions (CD, CM, and FW). The authors proposed that practitioners should carefully consider the higher acceleration and deceleration load imposed on wide players as compared to central players (Vigh-Larsen et al., 2018) as these actions were notably linked to neuromuscular fatigue in elite women soccer players (Douchet et al., 2021). This is due to the greater neural activation of working muscles, compared to constant-speed running (Mero and Komi, 1986). Playing position differences registered using absolute training loads are concordant with observed competitive positional demands. However, such a similarity cannot ensure that competitive demands are exactly reproduced due to the magnitude of the differences between the playing positions. Relative training loads aim to help practitioners program training in a way to reflect the real match demands (Guerrero-Calderón et al., 2022). Here, when accounting for relative training loads, markedly different conclusions emerged. For instance, while CD often previously report lower absolute training loads (Akenhead et al., 2016; Malone et al., 2015), results revealed that CD performed significantly greater relative training loads than most positions during the entire microcycle. Similarly, while WM reported the greatest competitive demands and weekly absolute values, relative values suggested that they were less likely to replicate competition demands. This result is consistent with observations in a previous study conducted in professional players (Baptista et al., 2019). These authors reported that the WM position was less likely to replicate its competitive demands as compared to the other playing positions. More specifically, WM are well known to perform numerous changes in speed during competitive matches (Vigh-Larsen et al., 2018). However, our results demonstrated that central positions (CD and FW) performed a significantly greater relative number of accelerations and decelerations than wide players. These competitive positional differences are not reproduced during training. Practitioners should consider relative training loads for optimal programming and pay greater attention to increasing the training load in wide players potentially through additional and specific work following the session (Guerrero-Calderón et al., 2022). Furthermore, the competitive demands are not reproduced to the same extent for all the GPS variables analysed during training. Indeed, a player exactly reproducing the competition demands would obtain 100% relative values during each single session (horizontal line in Figure 1, Figure 2, Figure 3, Figure4, Figure 5 and Figure 6). As can be seen from the various figures (except for moderate-speed distance and to a lesser extent accelerations and decelerations), this was evidently not the case. Indeed, data were far below 100% for the relative total, high-speed, and sprint distances covered throughout the microcycle. Of course, replicating the total distance of the match during training is impractical and not the main aim of practitioners. However, relative high-speed and sprint distances are much easier to replicate and are not time-constrained. Academy practitioners should consider increasing high-speed and sprint distances during the week to approach 100% relative training loads. Indeed, high-intensity efforts have continuously increased in elite soccer with these efforts more and more crucial to achieve competitive results (Di Salvo et al., 2009; Nassis et al., 2020). Moreover, high-intensity distance has been shown to differentiate across playing standards (Mohr et al., 2003). Increasing high-intensity distances covered in academy settings is necessary for the development of young players and to bridge the gap from academy to professional status; a training stimulus continuously below competitive demands will be inefficient to increase high-intensity distances during matches. High-velocity efforts could easily be increased with different field sizes during small-sided games (Riboli et al., 2020; Sangnier et al., 2019) or with additional game-specific drills (e.g., large-sided games) (Gabbett and Mulvey, 2013) or dissociated work (e.g., repeated 40m sprints) (Hauden et al., 2013; Iaia et al., 2009). Furthermore, high-intensity efforts are characterized by numerous accelerations and decelerations. The current results, and others, revealed that accelerations are extensively replicated during training (Clemente et al., 2019; Guerrero-Calderón et al., 2022) which is generally explained by the large use of small-sided games (Ade et al., 2014; Dalen et al., 2021). However, performing numerous accelerations and decelerations has been suggested to decrease the level of readiness (Douchet et al., 2021). Optimizing the balance between high-intensity distance and number of accelerations can be facilitated using relative training loads and help avoid unexpected fatigue. To the best of our knowledge, this study was the first to explore the differences between absolute and relative training loads in elite academy soccer players. Here, it was demonstrated that these quantification methods yield different conclusions. Using the relative method, WM performed the lowest weekly training load and CD the greatest. This study also demonstrated that training sessions fail to replicate competitive demands for most GPS-derived data. However, replicating competition during training sessions is not always easily achieved. As previously suggested, additional work after a training session is an alternative (Guerrero-Calderón et al., 2022). Studies should nevertheless be conducted to explore the effects of such supplementary work. A limitation here was that only a single academy soccer team was considered, with a limited number of matches. Accordingly, future research should replicate this study design in different contexts (e.g., senior players). In addition, future work could quantify relative training loads using individualized thresholds based on personalized physical capacities, to help improve training prescription and individualization.
The present results, obtained in academy soccer players, highlighted the need to quantify relative training loads to help contextualize training programming according to the competitive demands. This study challenges previous results demonstrating that WM perform greater training loads than other positions, and CD lower training loads using absolute thresholds. For instance, some GPS-derived data such as the relative moderate-speed distance revealed that WM were underloaded compared to other positions, while CD performed the greatest relative training loads. Furthermore, during the microcycle, these results demonstrated that competitive demands were only replicated for low-velocity thresholds, accelerations, and decelerations. The possible overuse of small-sided games (although evidently important for soccer performance) does not facilitate replication of high-velocity efforts. Practitioners in soccer academies, should therefore increase high-intensity demands over the week using, for example, large-sided games and/or dissociated drills including sprinting and high-intensity efforts at the end of training sessions.
ACKNOWLEDGEMENTS |
The Authors gratefully acknowledge participants and coaches of the Dijon Football Côte d’Or (DFCO). The authors have no conflicts of interest to declare. The results of the study are presented clearly, honestly, and without inappropriate data manipulation. The experiments comply with the current laws of the country in which they were performed. |
|
AUTHOR BIOGRAPHY |
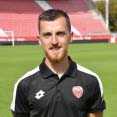 |
Tom Douchet |
Employment: PhD student in Sport Sciences, Dijon Football Côte d’Or (DFCO) and INSERM UMR1093-CAPS |
Degree: MSc |
Research interests: Soccer; periodization; youth |
E-mail: tom.douchet@gmail.com |
|
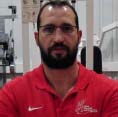 |
Christos Paizis |
Employment: INSERM UMR1093-CAPS, Professor at the Sport Sciences |
Degree: PhD |
Research interests: Motor control, biomechanics, neuroscience |
E-mail: christos.paizis@u-bourgogne.fr |
|
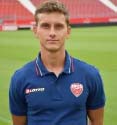 |
Hugo Roche |
Employment: Dijon Football Côte d’Or (DFCO) |
Degree: MSc |
Research interests: Soccer; periodization; youth |
E-mail: roche.hugo@hotmail.com |
|
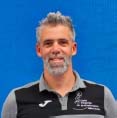 |
Nicolas Babault |
Employment: INSERM UMR1093-CAPS, Professor at the Sport Sciences |
Degree: PhD |
Research interests: Exercise training, neuromuscular system, electrical stimulation |
E-mail: nicolas.babault@u-bourgogne.fr |
|
|
|
REFERENCES |
 Ade J.D., Harley J.A., Bradley P.S. (2014) Physiological response, time-motion characteristics, and reproducibility of various speed-endurance drills in elite youth soccer players: Small-sided games versus generic running. International Journal of Sports Physiology and Performance 9, 471-479. Crossref
|
 Akenhead R., Harley J.A., Tweddle S.P. (2016) Examining the external training load of an english premier league football team with special reference to acceleration. Journal of Strength and Conditioning Research 30, 2424-2432. Crossref
|
 Akenhead R., Hayes P.R., Thompson K.G., French D. (2013) Diminutions of acceleration and deceleration output during professional football match play. Journal of Science and Medicine in Sport 16, 556-561. Crossref
|
 Akenhead R., Nassis G.P. (2016) Training load and player monitoring in high-level football: Current practice and perceptions. International Journal of Sports Physiology and. Performance 11, 587-593. Crossref
|
 Baptista I., Johansen D., Figueiredo P. (2019) Positional Differences in Peak- and Accumulated- Training Load Relative to Match Load in Elite Football. Sports 8. Crossref
|
 Bradley P.S., Sheldon W., Wooster B., Olsen P., Boanas P., Krustrup P. (2009) High-intensity running in English FA Premier League soccer matches. Journal of Sports Sciences 27, 159-168. Crossref
|
 Chmura P., Liu H., Andrzejewski M., Chmura J., Kowalczuk E., Rokita A., Konefał M. (2021) Is there meaningful influence from situational and environmental factors on the physical and technical activity of elite football players? Evidence from the data of 5 consecutive seasons of the German Bundesliga. Plos One 16, 1-16. Crossref
|
 Clemente F.M., Rabbani A., Conte D., Castillo D., Afonso J., Clark C.C.T., Nikolaidis P.T., Rosemann T., Knechtle B. (2019) Training/match external load ratios in professional soccer players: A full-season study. International Journal of Environmental Research and Public Health 16. Crossref
|
 Cohen, J. (1988) Statistical power analysis for the behavioral sciences. 2nd ed. ed. Lawrence Erlbaum Associates. Crossref
|
 Dalen T., Sandmæl S., Stevens T.G.A., Hjelde G.H., Kjøsnes T.N., Wisløff U. (2021) Differences in Acceleration and High-Intensity Activities Between Small-Sided Games and Peak Periods of Official Matches in Elite Soccer Players. Journal of Strength and Conditioning Research 35, 2018-2024. Crossref
|
 Di Salvo V., Baron R., Tschan H., Calderon Montero F.J., Bachl N., Pigozzi F. (2007) Performance characteristics according to playing position in elite soccer. International Journal of Sports Medicine 28, 222-227. Crossref
|
 Di Salvo V., Gregson W., Atkinson G., Tordoff P., Drust B. (2009) Analysis of high intensity activity in premier league soccer. International Journal of Sports Medicine 30, 205-212. Crossref
|
 Douchet T., Humbertclaude A., Cometti C., Paizis C., Babault N. (2021) Quantifying Accelerations and Decelerations in Elite Women Soccer Players during Regular In-Season Training as an Index of Training Load. Sports 9, 109. Crossref
|
 Gabbett T. J., Mulvey M.J. (2013) Time-motion analysis of small-sided training games and competition in elite women soccer players. Journal of Strength and Conditioning Research 22, 543-552. Crossref
|
 Guerrero-Calderón B., Fradua L., Morcillo J.A., Castillo-Rodríguez A. (2022) Analysis of the Competitive Weekly Microcycle in Elite Soccer: Comparison of Workload Behavior in Absolute and Relative Terms. Journal of Strength and Conditioning Research 37, 343-350. Crossref
|
 Hauden T.A., Tønnessen E., Hisdal J., Seiler S. (2013) The Role and Development of Sprinting Speed in Soccer. International Journal of Sports Physiology and Performance 9, 432-441. Crossref
|
 Iaia M.F., Rampinini E., Bangsbo J. (2009) High-intensity training in football. International Journal of Sports Physiology and Performance 4, 291-306. Crossref
|
 Impellizzeri F.M., Marcora S.M., Coutts A.J. (2019) Internal and external training load: 15 years on. International Journal of Sports Physiology and Performance 14, 270-273. Crossref
|
 Malone J.J., Di Michele R., Morgans R., Burgess D., Morton J.P., Drust B. (2015) Seasonal training-load quantification in elite English Premier League soccer players. International Journal of Sports Physiology and Performance 10, 489-497. Crossref
|
 Mero A., Komi P. V. (1986) Force-, EMG-, and elasticity-velocity relationships at submaximal, maximal and supramaximal running speeds in sprinters. European Journal of Applied Physiology and Occupational Physiology 55, 553-561. Crossref
|
 Modric T., Jelicic M., Sekulic D. (2021) Relative training load and match outcome: Are professional soccer players actually undertrained during the in-season?. Sports 9. Crossref
|
 Mohr M., Krustrup P., Bangsbo J. (2003) Match performance of high-standard soccer players with special reference to development of fatigue. Journal of Sports Sciences 21, 519-528. Crossref
|
 Nassis G.P., Massey A., Jacobsen P., Brito J., Randers M.B., Castagna C., Mohr M., Krustrup P. (2020) Elite football of 2030 will not be the same as that of 2020: Preparing players, coaches, and support staff for the evolution. Scandinavian Journal of Medicine and Science in Sports 30, 962-964. Crossref
|
 Owen A.L., Lago-Penñs C., Gómez M.Á., Mendes B., Dellal A. (2017) Analysis of a training mesocycle and positional quantification in elite European soccer players. International Journal of Sports Science and Coaching 12. Crossref
|
 Riboli A., Coratella G., Rampichini S., Ce E., Esposito F. (2020) Area per player in small-sided games to replicate the external load and estimated physiological match demands in elite soccer players. Plos One 15, 1-15. Crossref
|
 Sangnier S., Cotte T., Brachet O., Coquart J., Tourny C. (2019) Planning Training Workload in Football Using Small-Sided Games’ Density. Journal of Strength and Conditioning Research 33, 2801-2811. Crossref
|
 Vigh-Larsen J., Dalgas U., Andersen T. (2018) Position-specific acceleration and deceleration profiles in elite youth and senior soccer players. Journal of Strength and Conditioning Research 32, 1114-1122. Crossref
|
 Willmott A.G.B., James C.A., Bliss A., Leftwich R.A., Maxwell N.S. (2019) A comparison of two global positioning system devices for team-sport running protocols. Journal of Biomechanics 83, 324-328. Crossref
|
 Zurutuza U., Castellano J., Echeazarra I., Casamichana D. (2017) Absolute and relative training load and its relation to fatigue in football. Frontiers in Psychology 8, 1-8. Crossref
|
|
|
|
|
|
|